Ibrahim Hemadesh*1; Farajollah Shahriari1; Mohammad Farsi1
1, Department of Biotechnology and Plant Breeding, Faculty of Agriculture, Ferdowsi University of Mashhad, Iran
E-mail:
Ibrahim55@gmail.com
Received: 14/11/2019
Acceptance: 25/11/2019
Available Online: 23/02/2020
Published: 01/04/2020
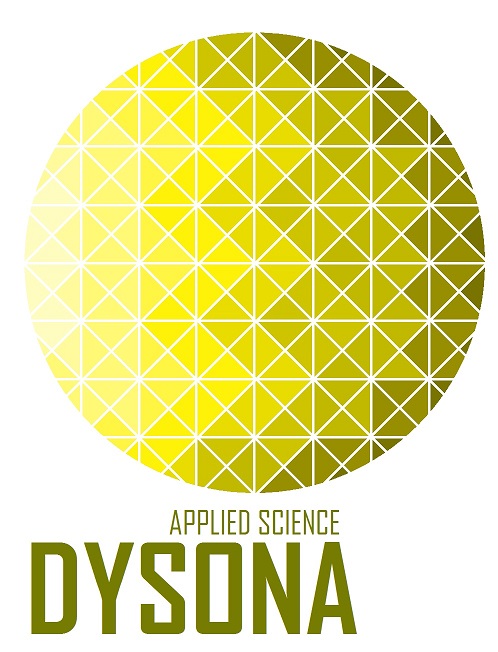
Manuscript link
http://dx.doi.org/10.30493/das.2020.103715
Abstract
Polyploidy induction can be used as a method to improve forage quality and crop yield. Additionally, autopolyploidy may play a key role in tolerance pathways of biotic and abiotic stresses, such as nutrient deficiency, drought, extreme temperatures, pests, and pathogens. Colchicine is widely used as a polyploidy inducer in plant species. To examine this ability in forage sorghum, the shoot meristems of four-day-old seedlings of “Omid-Bakhsh” sorghum cultivar were treated with colchicine. Three levels of colchicine concentration (0, 0.01, 0.1, and 0.2) and four-time periods (4, 8, 16, and 24 hours) were used. Morphological and cytological properties of treated and non-treated plants were assessed. Results showed that the polyploidy plants had a lesser height, leaf length and number of stomata compared to the normal plants; however, leaf width and thickness, stem diameter, pollen diameter, as well as stomatal length and width were significantly higher in polyploidy plants. On the other hand, there was no significant difference between treated plants and control in regards to the number of leaves. Furthermore, the highest probability of polyploidy induction rate in “Omid-Bakhsh” cultivar was observed at 0.2% colchicine concentration and eight hours treatment as revealed by flow cytometry data. Protein analysis results showed a significant increase in protein content of polyploidy plants compared to control.
Keywords: Polyploidy, morphological Characteristics, Forage Sorghum, Colchicine
Introduction
Polyploidy is occurred when two or more extra sets of the basic chromosomes are added in an organism. Autopolyploid is referred to an organism with duplication of full genome of a species [1]. Nowadays, chemical agents such as colchicine, oryzalin, taxol, vincristine, vinblastine, vinorelbine, cryptophycin, and trifluoride are used to disturb mitosis and induce polyploidy. The effect of colchicine on mitosis was first studied in mice, but its successful use in polyploidy induction was reported for the first time by Blakeslee in 1937 [2]. Subsequently, Blacksley reported successful polyploidy induction using colchicine in 48 species belong to 29 different genus and 16 families of flowering plants. One of the major advantages of colchicine treatment is related to its application in autoploidization of diploid plants.
One of the rapid and most widespread effects of polyploidy on plants is to stimulate the growth of vegetative organs and thus more yield than counterpart diploid plant [3]. Furthermore, when compared to their diploid counterpart, polyploid plants tend to have thicker, wider and shorter leaves and petiole texture, in addition to shorter shoots, slower flowering, late flowering, reduced pollen fertility and seed set formation [4]. Another general effect of polyploidy is to increase cell, stomata and pollen grain size. These aspects are often used as a marker for detection of polyploidy level among mixed populations or herbarium specimens. In a study conducted on Citrus limonia, it was found that leaves of tetraploid plants were significantly thicker than the diploid one, mainly due to their thick spongy parenchyma with large intercellular spaces and epidermal cells [5]. Also, polyploidy at different levels can have a wide range of effects on plants, including larger flower and fruits, extended flowering time, overcoming hybridization barriers, a decrease or completely inhibit seed set production, enhancing pests resistance and stress tolerance [6,7]. Studies have also shown that artificial tetraploidy in plants can lead to increased biomass and phytochemicals production [8].
Forage sorghum (Sorghum bicolor L. Moench) a member of the Gramineae family is a species consisted of several types and variants with the genetic formula of 2n=2x=20. Plants within of this species are short-day, four-carbon with high-photosynthetic performance and most of their varieties need temperatures above 21 degrees Celsius to maintain a proper growth rate. One of the main aspects of forage sorghum is its tolerance drought compared to other grains. Furthermore, it is adapted with a wide variety of soils [9].
By increasing of demand for milk and meat products and considering the previously mentioned aspects, the importance of sorghum as a worldwide forage crop especially in Asia is quite clear [10]. However, in order to meet the increased demand for forage crops especially when considering nowadays challenges such as the rapid population growth and the reduced cropping areas, new sorghum cultivars with high yields and biomass have to be bred. Therefore, in the present study, the main goal was to induce polyploidy by using different concentrations of colchicine to investigate the possibility of a chromosomal duplication of diploid sorghum and compare their morphological characteristics of the obtained polyploidy with its diploid counterpart.
Materials and Methods
Plant material
In order to study the effects of polyploidy induction on forage sorghum seeds, a commercial forage sorghum line (Omid-Bakhsh MSF8) was provided from Khorasan Razavi Agricultural and Natural Resources Research Center. The experiment was conducted in a completely randomized design with two factors including three levels of colchicine concentrations (0, 0.01, 0.1 and 0.2%) and three periods of time (4, 8, 16 and 24 hours) with three replications which was imposed on four-day-old seedlings meristems. Seedlings were immersed in 5 ml tubes containing colchicine solution in an upside-down position while the roots were in contact with moist cotton. Treated seedlings were rinsed with distilled water and gradually transferred to the greenhouse. The soil composition was normal soil and sand (1: 1). For this purpose, 5 kg plastic pots were used. Control plants were also cultivated under these conditions. Irrigation and all proper agricultural practices were performed uniformly for all pots until the end of the experiment. Survival rate was evaluated in treated seedlings after one month of the treatment.
Plant traits screening
Plant height was recorded as the length from the soil surface to the tip of the cluster. Three leaves (second, third and fourth leaves) were used to measure leaf length, width, and thickness. [11].
To determine the number of leaf surface stomata, two fully developed leaves of the same size and age were selected from the middle part of each plant. For this purpose, the epidermis of the underside of the leaves was treated with a thin layer of ordinary colorless nail polish diluted with acetone. After the solution was dried, the top layer was removed using tape and mounted on a clean microscopic slide. The optical microscope was used to observe and count the number of apertures per square millimeter. Stomata length, width and density index are commonly used as a method for identifying polyploidy plants from their counterpart diploid plants [12][13].
Stomata dimensions were measured by 10X magnification. Considering the stomata inconsistencies in dimensions and density especially around the veins, the samples were rotated under the microscope in order to take different photos for each. Images were processed and evaluated for length, width and density (number of stomata/image area) of the stomata using ImageJ software in comparison to a known length [14].
To study pollen diameter, several anthers were sampled from each of the treated and control plants. Anthers were then stored in absolute ethanol and acetic acid (1: 3) for 24 h and subsequently in 70% ethanol. Anthers pollen were stained with a 2% aceto-orcein solution over lamellae and investigated under the microscope with 40X magnification [15].
Flow cytometry assay
Ploidy level of plants was characterized using a (Partec PA, Germany) flow cytometer. Control plants were also examined to compare the effects of colchicine, and the peak obtained from them was the basis of the sample volume comparisons. Samples of one squared centimeter were taken from young and mature leaves (approximately two months old) and one milliliter of Galbraith extraction buffer was added to each sample [16]. A razor blade was used to shred the tissue inside the buffer solution without crushing cells. The suspension of plant DNA was then passed through the flow cytometer’s filtrate and one ml of kernel staining solution containing 4 µl of DNA solution was added subsequently. The solution was then centrifuged at 4 ○C for 10 minutes and 10000 rpm and the resultant supernatant was injected. For each sample, the volume of 10,000 nuclei was measured and the obtained peaks were interpreted using Motfid software.
Protein content
To extract protein from plant tissue Tris-HCl method was used [17]. Samples of one gram of sorghum leaf were powdered in liquid nitrogen. The resulting herbal powder was mixed directly with 3 ml Tris-HCl buffer (100 mM Tris-HCl aqueous solution, 10 mM EDTA pH = 8, 0.5% beta-mercaptoethanol, 50 mM PMSF) which has been precooled in ice. After centrifugation at 11000 g for 20 minutes at 4 °C, the supernatant was removed and used for the assay. To prepare the stock solution, 0.1 g of Coomassie Brilliant Blue G-250 was dissolved in 50 ml of ethanol and after passing through filter paper, 100 ml of 85% phosphoric acid was added and distilled water was added until a volume of 200 ml. The resulting solution was kept in a dark container at 4 °C. To prepare the assay solution, one unit of color stock solution diluted with four units of distilled water was used for testing. To prepare bovine serum albumin (BSA) standard solution, 0.1 g of bovine serum albumin protein was dissolved in 1000 ml extraction buffer to reach a final concentration of 100 µg / ml. for each assay 60 µL of the extracts were added to 940 µL of assay solution and after 5 minutes, its absorbance was measured at 595 nm and compared against (BSA) standard curve.
Statistical analysis
Analysis of data was performed using JMP statistical software. Differences between survival rates related to each treatment were evaluated using Chi-square. The comparison between treatments means was performed using Student’s t-test at 5% level of probability.
Results and Discussion
Survival rates
The results of Chi-square test showed a significant difference between survival rates (p-value <0.0001). Mortality rates increased with the increase of colchicine concentration. The comparison of colchicine concentration-effect regardless of treatment duration showed that the lowest survival rate was observed in 0.2% concentration (45.83%). Furthermore, seedling survival decreased by increasing periods of time. These results are in agreement with those of reported by Elyazid and El-Shereif [18]. The low meristem survival rates were also reported by Sanford [6], under conditions that the concentration of colchicine solution was high or treatment period was too long. Colchicine activity appears to be concentrated in the meristem, causing a physiological disturbance, a reduction of cell division or explant death [19].
Morphological screening
Possible polyploidy plants were screened based on changes in morphological characteristics. The first morphological change observed in the treated plants was that the preliminary leaves had an abnormal appearance, whereas the later leaves had a natural appearance compared to the control. This phenomenon may be due to stress imposed by colchicine treatment. This type of rosette formation was reported by [20] in the genus Sorghum after two to three weeks of germination.
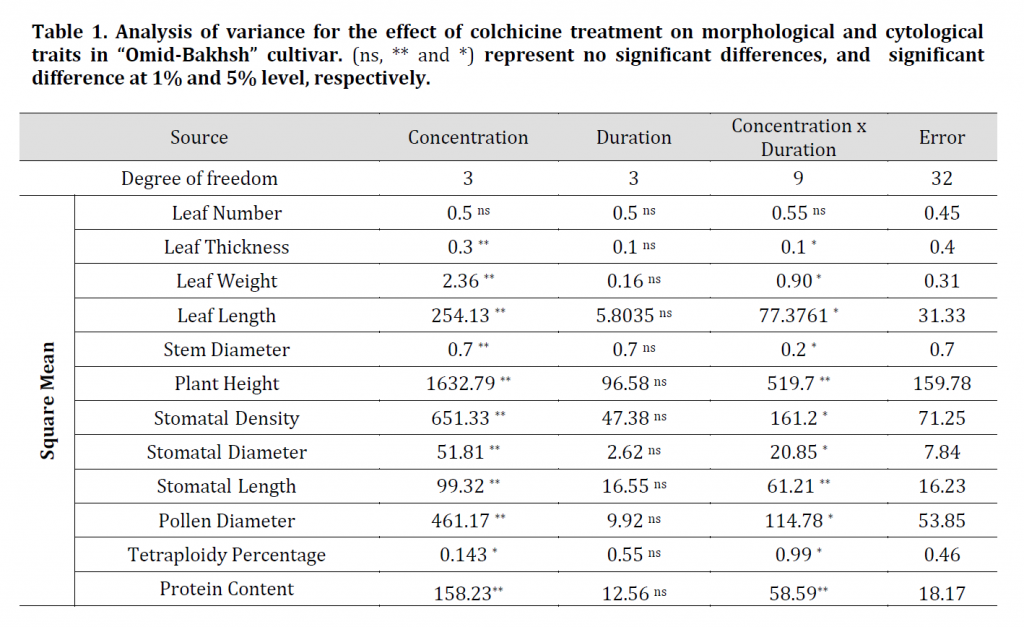
Evaluation of plant height data showed that all concentrations of colchicine had a significant effect on height (Table 1); however, there was no significant difference between different concentrations of colchicine on plant height. The lowest plant height was observed with treatment of 0.2% colchicine with an 8-hour time period of (113.43 cm) and the highest plant height in control (163.16 cm) which might render colchicine treated plant as a more convenient silage production source. An increase in stem diameter was observed by increasing colchicine concentration. The highest stem diameter was recorded at 8 h and 0.2% colchicine treatment (0.92 cm) while the control scored the lowest stem diameter (0.57 cm). Morphological and apparent features such as stem length and diameter have been cited as indirect, easy, fast and reliable symptoms of ploidy identification, but require time for plant growth and are less robust than direct methods [21]. As an example, the results of the study by [22], on ‘Cape periwinkle’ (Catharanthus roseus cv. Alba), showed that by increasing of ploidy level, the stem diameter of plants is increased. Furthermore, plant height of tetraploid plants was significantly lower than diploid ones.
Results of analysis of variance on the effect of colchicine on leaf length, width, and thickness showed that there was a significant difference between treated and control plants at 1% probability level; however, no significant difference was observed for leaf number (Table 1). Although the mean leaf length showed a significant difference between the treated and control plants, no significant difference was observed between the plants treated with different concentrations of colchicine. Colchicine decreased the leaf length of treated plants in comparison to control plants. Both leaf weight and thickness were significantly increased in colchicine treated plants compared to control plants (Table 2). Similar results were obtained with basil [23] and Salvia [26] where tetraploid plants an increased leaf thickens.
Stomatal indices
Since stomatal size and number is related to the number of chromosomes, rapid counting in addition to stomatal measurements are considered valuable for screening ploidy levels [23][24][25]. The results of stomatal characteristics data analysis of treated and control plants showed significant differences for stomatal length, width, and density (Table 1). The highest stomatal length and width were observed at 0.2% concentration with means of 37.22 and 23.74 μm, respectively while the lowest in control plants with means of 30.29 and 18.70 μm, respectively. By Increasing of colchicine concentration, the number of stomata in leaf area was decreased. The lowest value was observed in 0.2% of colchicine with a mean of 45.75 stomata/mm2 and the highest value in control with a mean of 63.08 stomata/mm2. These results are similar to those in [27] with Anthurium, [28] with sorghum and [26] with salvia, where stomatal density was decreased in plants treated with an increasing concentration of colchicine. Furthermore, both Hosseini and chen reported an increase in stomatal size in the treated plants compared to control [27] [26]. Studies have shown that the size of stomatal guard cells is more influenced by genetic factors and less affected by environmental conditions than other plant cells. As the ploidy level increases, the length and width of the stomata increase, leading to the reduction of stomatal density [29]. Therefore, it is inferred that the evaluation of stomatal indices can play a crucial role in characterization of different ploidy levels.
Pollen size
According to the results, pollen grain diameter was significantly affected by colchicine treatment (Table 1). The highest pollen diameter was observed in 8 hours with 0.2% colchicine (mean value of 69.35 μm) and the lowest pollen diameter was recorded in the control plants with a mean of 45.33 μm (Table 2). These results are in agreement with the results of [30] on Torenia fournieri where tetraploid plants had larger pollen grains.
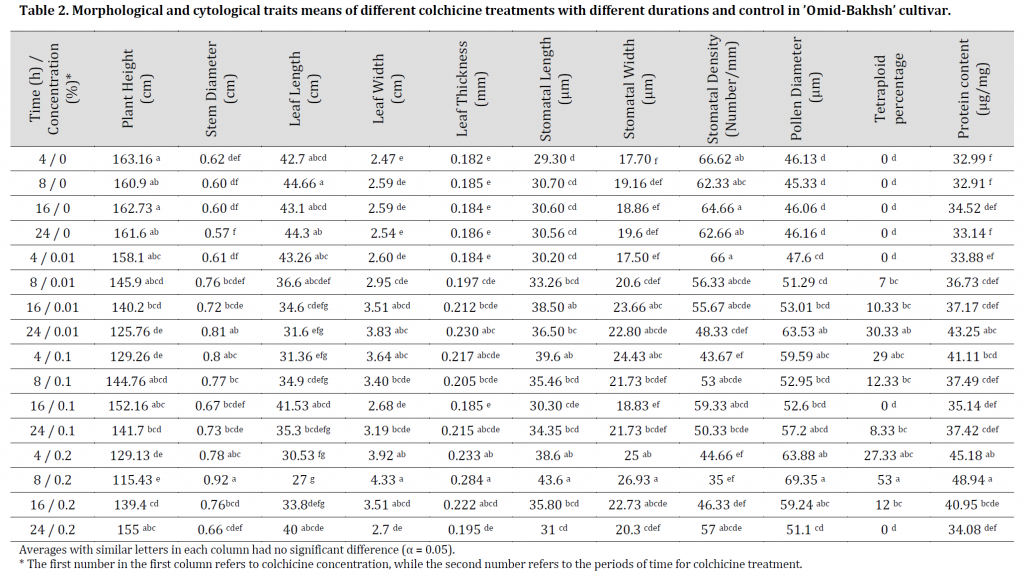
Flow cytometry assay
Ploidy levels were assayed using flow cytometry technique by comparing colchicine treated samples with control. Diploid plants were applied as standard specimens with two 1G and 2G peaks averaging 13.78 and 26.93 (Fig. 1)(A). Therefore, the level of DNA ploidy in these plants is 2X=2C. Flow cytometric histograms of seedlings treated with colchicine 1G and 2G peaks showed averages of 26.13 and 52.26 (Fig. 2)(B), which refers to their tetraploidy level with an estimation of 4X=2C. Furthermore, the histograms obtained from mixoploid plants also have three peaks with mean values of 13.79, 26.83 and 52.58 (Fig. 1)(C).
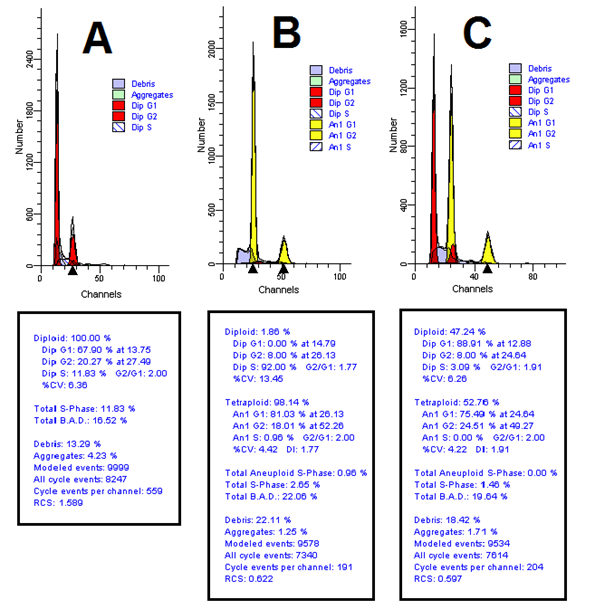
Results of analysis of variance based on flow cytometric data showed that the effect of colchicine on tetraploid percentage was significant at 5% probability level. As the concentration of colchicine increased from 0 to 0.2%, the tetraploid percentage increased from 0 to 23%. It should be noted that there was no significant difference between 0.01 and 0.1 levels of colchicine concentration. (Fig. 2).
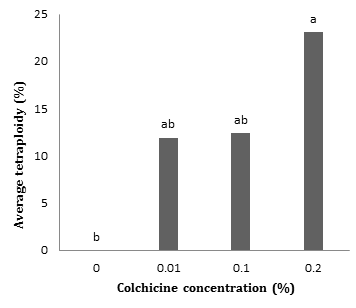
The effect of colchicine treatment imposed at different periods of time was not significant on tetraploid percentage (Table 1). However, their interaction was significant at 5% probability level as the highest tetraploid percentage was observed in 8 h treatment with 0.2% concentration, 24 h with 0.01% concentration and 4 h with 0.1%, respectively (Table 2).
The results of flow cytometry showed that colchicine treatment has an ability to affect most of the treated seedlings and while some plants reached a nearly pure tetraploid percentage of 98%, which is close to the pure tetraploid, the rest of the plants had different tetraploid percentages. Three levels of ploidy were observed including diploid (2X), tetraploid (4X), and mixoploid (2X+4X). Since the central region of meristem is responsible for the production of stem end cells, duplication of chromosomes in this area generates terminal polyploidy tissue. It appears that pure polyploidy plants result from the successful and appropriate doubling of cells treated in all tissue layers of the central region in the meristematic area, which eventually produce plants with homogeneous tetraploid cells [31]. The creation of mixoploid plants is not unexpected since the meristem of the plants is composed of many tissue layers with multiple cells and may have a tendency to absorb colchicine [32] [33]. Other factors might be involved in the production of mixoploid plants such as the differential and un-equal effect of colchicine on cell dividing [34]. Tetraploid impurities were also reported in other studies such as [35] on Melissa officinalis and [36] on Narcissus.
Protein content
Results showed that colchicine treatment significantly Increased protein content in the resulted plants with no significant effect for the treatment duration. Furthermore, the interaction between treatment time and concentration on total protein content was significant (Table 1). The highest protein content was observed in plants treated with 0.2% colchicine for 8 hours (48.94 µg/mg) while the lowest was observed in control plants (32.91 µg/mg). These results are similar to [19] results on the endosperm protein content of autopolyploid rice lines when compared to diploid ones. This increase in protein content might be contributed to the reported gene expression increase in polyploid plants compared to diploid plants [37].
Conclusions
As a conclusion, colchicine treatment affected the morphological traits of “Omid-Bakhsh” forage sorghum cultivar resulting in a decrease in stem length and leaf length, with an increase in stem diameter, leaf width, leaf thickness, and protein content. However, no changes in leaf number were observed. The application of higher colchicine concentrations resulted in an increase in stomatal dimensions and pollen diameter with a decrease in stomatal density. The results of flow cytometry data showed that the highest probability of polyploidy in “Omid-Bakhsh” cultivar was contributed to 0.2% colchicine treatment and duration of 8 hours. These findings are considered promising as a starting point of introducing new tetraploid forage sorghum types with compacted stems and higher protein content.
References
1 | Chen ZJ. Molecular mechanisms of polyploidy and hybrid vigor. Trends Plant Sci. 2010;15(2):57-71. DOI |
2 | Blakeslee AF, Avery AG. Methods of inducing doubling of chromosomes in plants: by treatment with colchicine. J. Hered. 1937;28(12):393-411. DOI |
3 | Roy QJ. Sorghum improvement and the genetics of growth. Texas A&M University Press; 1974. |
4 | Farsi, M., Ghaboli, M., Mahmoudnia, M., Plant Cytogenetics, Ferdowsi University of Mashhad Publications; 2009, PP:137-140-144. |
5 | Allario T, Brumos J, Colmenero-Flores JM, Tadeo F, Froelicher Y, Talon M, Navarro L, Ollitrault P, Morillon R. Large changes in anatomy and physiology between diploid Rangpur lime (Citrus limonia) and its autotetraploid are not associated with large changes in leaf gene expression. J. Exp. Bot. 2011;62(8):2507-19. DOI |
6 | Sanford JC. Ploidy manipulations. Methods in fruit breeding. 1983:100-23. |
7 | Predieri S. Mutation induction and tissue culture in improving fruits. Plant Cell Tissue Organ Cult. 2001;64(2-3):185-210. DOI |
8 | Wallaart TE, Pras N, Quax WJ. Seasonal variations of artemisinin and its biosynthetic precursors in tetraploid Artemisia annua plants compared with the diploid wild-type. Planta Med. 1999;65(08):723-8. DOI |
9 | Doggett H. Tetraploid varieties of Sorghum vulgare. Nature. 1957;179(4563):786. DOI |
10 | Arti P, Devi DR, Murty UR. Embryogenic callus induction and plant regeneration in forage sorghum Sorghum biocolor (L.) Moench. Cereal Res. Commun. 1994;1:71-7. |
11 | Saedi, M., Honarmand S.G., Resayi, A. Plant breeding through physiological traits, Ferdowsi University of Mashhad Publications; 2015, P:185. |
12 | Saharkhiz, MJ. The effects of some environmental factors and ploidy level on morphological and physiological characteristics of feverfew (Tanacetum parthenium L.) medicinal ornamental plant. PhD dissertation, Tarbiat Modares University, Tehran, 2007. |
13 | Yaroyi, p. Evaluation of the effect of colchicine on morphological, physiological and effective materials characteristics of medicinal plant (Dracocephalum moldavica L.) M.Sc.Thesis Tarbiat Modares University, Tehran, 2007. |
14 | Hamill SD, Smith MK, Dodd WA. In vitro induction of banana autotetraploids by colchicine treatment of micropropagated diploids. Aust. J. Bot. 1992;40(6):887-96. DOI |
15 | Funamoto T, Kondo K, Tatarenko IV, Gontcharov A, Verkholat VP, Smirnov SV. Intraspecific polyploidy of Parnassia palustris var. multiseta (Saxifragaceae sl) collected in Primorye and Altai Territories, Russia. Chromosom Bot. 2006;1(1):23-6. DOI |
16 | Galbraith DW, Harkins KR, Maddox JM, Ayres NM, Sharma DP, Firoozabady E. Rapid flow cytometric analysis of the cell cycle in intact plant tissues. Science. 1983;220(4601):1049-51. DOI |
17 | Bradford MM. A rapid and sensitive method for the quantitation of microgram quantities of protein utilizing the principle of protein-dye binding. Anal. Biochem. 1976;72(1-2):248-54. DOI |
18 | Elyazid DM, El-Shereif AR. In vitro induction of polyploidy in Citrus reticulata Blanco. Am J Plant Sci. 2014;5(11):1679. DOI |
19 | Chen ZJ. Genetic and epigenetic mechanisms for gene expression and phenotypic variation in plant polyploids. Annu. Rev. Plant Biol.. 2007;58:377-406. DOI |
20 | Franzke CJ, Ross JG. Colchicine induced variants in sorghum. J. Hered. 1952;43(3):107-15. DOI |
21 | Sari N, Abak K, Pitrat M. Comparison of ploidy level screening methods in watermelon: Citrullus lanatus (Thunb.) Matsum. and Nakai. Sci. Hortic. 1999;82(3-4):265-77. DOI |
22 | Hosseini, H., Chehrazi, M., Nabati Ahmadi, D., Mahmmodi Sorestani, M. Effect of colchicine treatment on the autotetraploidy induction and morpho-physiological traits alteration in Catharanthus roseus cv. alba. Plant Production Technology. 2014;5(2): 55-62. |
23 | Omidbaigi R, Mirzaee M, Hassani ME, Sedghi Moghadam M. Induction and identification of polyploidy in basil (Ocimum basilicum L.) medicinal plant by colchicine treatment. Int J Plant Prod. 2012;4(2):87-98. DOI |
24 | Sarvella P, Meyer JR, Owings A. A Scotch Tape Method for Counting or Measuring Stomata 1. Crop Science. 1961;1(1):81-2,. |
25 | Gao SL, Chen BJ, Zhu DN. In vitro production and identification of autotetraploids of Scutellaria baicalensis. Plant Cell Tissue Organ Cult. 2002;70(3):289-93. DOI |
26 | Hosseini, B., Javanbakht, S. Effects of in vitro polyploidy induction on some morphological, physiological and biochemical traits of Salvia leriifolia Benth. Tahqiqati Zhinitik Islahi Giyahani Martaii Jangalii Iran. 2017;25(1). |
27 | Chen C, Hou X, Zhang H, Wang G, Tian L. Induction of Anthurium andraeanum “Arizona” tetraploid by colchicine in vitro. Euphytica. 2011;181(2):137-45. DOI |
28 | Vanitha J, Jiang S, Ramachandran S. Impact of Colchicine Treatment on Sorghum bicolor BT×623. Mol Plant Breed. 2013;4. DOI |
29 | Watrous SB, Wimber DE. Artificial induction of polyploidy in Paphiopedilum. Lindleyana. 1988;3(4):177-83. |
30 | Tandon SL, Bhutani K. Morphological and cytological studies of colchicine-induced tetraploids inTorenia fournieri lind. Genetica. 1965;36(1):439-45. |
31 | Jones JR, Ranney TG, Eaker TA. A novel method for inducing polyploidy in Rhododendron seedlings. J. Amer. Rhododendron Soc. 2008;62(3):130-5. |
32 | Koutoulis A, Roy AT, Price A, Sherriff L, Leggett G. DNA ploidy level of colchicine-treated hops (Humulus lupulus L.). Sci. Hortic. 2005;105(2):263-8. DOI |
33 | Tambong JT, Sapra VT, Garton S. In vitro induction of tetraploids in colchicine-treated cocoyam plantlets. Euphytica. 1998;104(3):191-7. DOI |
34 | Wan Y, Petolino JF, Widholm JM. Efficient production of doubled haploid plants through colchicine treatment of anther-derived maize callus. Theor. Appl. Genet. 1989;77(6):889-92. DOI |
35 | Borgheei SF, Sarikhani H, Chaichi M, Kashi A. In vitro induction of polyploidy in lemon balm (Melissa officinalis L.). Iran. J. Med. Aromat. Plants. 2010;26(3). |
36 | Ahmadi MM, Sarikhani H, Chaichi M, Kashi A. An Investigation in to Micropropagation and the Effect of Colchicine on In Vitro Ploidy Induction in Narcissus (Narcissus tazetta). Journal of Plant Production Agronomy, Breeding and Horticulture. 2013;35(4). |
37 | Osborn TC, Pires JC, Birchler JA, Auger DL, Chen ZJ, Lee HS, Comai L, Madlung A, Doerge RW, Colot V, Martienssen RA. Understanding mechanisms of novel gene expression in polyploids. Trends in genetics. 2003;19(3):141-7. DOI |
Cite this article:
Hemadesh, I., Shahriari, F., Farsi, M. Evaluation of tetraploid induction in forage sorghum cultivar “Omid-Bakhsh” using colchicine treatment. DYSONA – Applied Science, 2020;1(1): 1-10. doi: 10.30493/das.2020.103715