Michael Alabboud 1,2; Ali Javadmanesh 3
1, Department of Horticulture, Al-Baath University, Homs, Syria
2, Department of Horticultural Sciences, UTCAN, University of Tehran, Iran
3, Department of Animal Science, Faculty of Agriculture, Ferdowsi University of Mashhad, Mashhad, Iran
E-mail:
Alabboud@ut.ac.ir
Received: 08/04/2020
Acceptance: 16/04/2020
Available Online: 16/04/2020
Published: 01/07/2020
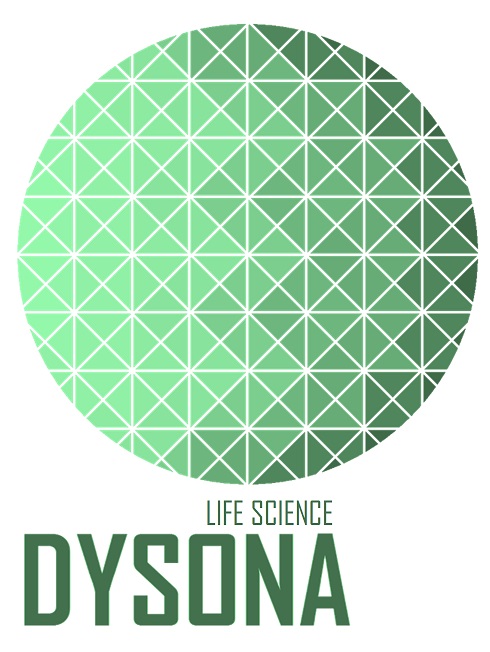
Manuscript link
http://dx.doi.org/10.30493/dls.2020.225404
Abstract
The novel coronavirus (SARS-CoV-2) has without a doubt escalated to become a global crisis. Taking into consideration our limited knowledge regarding the virus, all the efforts to provide better understanding or explore the solutions are highly welcomed. In this article, 88 conventional drugs, 16 vitamins, and 63 natural (plant) compounds were chosen to perform a binding simulation with the reported COVID-19 main protease (Mpro) in search for probable inhibitors. Based on docking results, various vitamins (B9, A, K, and E vitamins) exhibited a significantly strong interaction with the studied receptor which might refer to the importance of these supplements in daily diets. Additionally, the strong ligand-protein interactions of some conventional drugs such as Pleconaril, Adefovir dipivoxil, and Stavudine in addition to plant-based compounds such as Curcumin (Curcuma longa), Anolignan A (Anogeissus acuminata), and Phyllamyricin B (Phyllanthus myrtifolius) render these compounds promising and recommended for further studies.
Keywords: COVID-19, Mpro, Antiviral, Molecular docking
Introduction
The novel coronavirus (SARS-CoV-2) was originally discovered and spread in Wuhan, China in late 2019 and since then, the disease caused by this β-coronavirus was named by the world health organization (WHO) as coronavirus disease 2019 (COVID-19) [1]. The transmission factors for the disease are still debatable; however, the reservoir of the novel virus is thought to be bats [2] as a huge diversity of coronaviruses is found in them and therefore considered natural reservoirs of SARS-like coronaviruses [3]. While on the other hand, the infectious host from which the virus was originally transmitted to humans is still unknown [1] if there is any.
Many foggy points still surround the outbreak; however, the solid facts are that while the major percentage of COVID-19 cases of had developed mild flu-like symptoms, the rest few suffer from a rapidly developed acute respiratory distress syndrome (ARDS) which might lead to respiratory failure, in addition to multiple organ failure, and even death [4]. Additionally, the long incubation period 3–7 days with no clear evidence of the disease and capability of spreading the infection [5][6] have rendered the virus among the most dangerous especially for older patients and those of major underlining health conditions [1]. Thereafter, and based on the rapid increase in COVID-19 confirmed cases outside of China, WHO announced it a pandemic on 11 March 2020 [7].
SARS-CoV-2 genome encodes different structural and nonstructural proteins. The viral spike (S) protein is the main structural protein in cell invasion process as it facilitates the engagement with angiotensin-converting enzyme 2 (ACE2) of the host cell [8][1] and mediates the viral invasion by priming with host cell Transmembrane protease serine 2 (TMPRSS2) [8] which illustrate the importance of these components in drug design for the pandemic. There are components with the potential of strong binding to the viral spike protein or ACE2 [9][10]. These components could act as molecular blockers for disabling the virus’s ability to enter the cell. On the other hand, the nonstructural main protease (Mpro) facilitates the proteolytic processing of polyproteins and therefore controls the viral gene expression and replication processes which renders it an interesting target for drug design researches [11][12].
Since the original breakout, the efforts to develop an efficient vaccine and pharmaceutical therapies have been rapidly accelerated. Various drugs are already being tested in clinical trials with many showing promising results such as Chloroquine phosphate [13][14][15], Remdesivir [15], in addition to Hydroxychloroquine and Azithromycin [16]. On the other hand, traditional herbal medicine was used before to treat similar breakouts such as SARS and H1N1 which might render herbal extracts and substances as an alternative approach in COVID-19 treatment [17] especially that many of these extracts and compounds are already being used in the treatment of some chronic diseases such as diabetes, HIV/AIDS, and herpes [18][19][20]. The diversity in medicinal plants and their active products demands a rapid evaluation of the possible viral inhibitory effectiveness which might be assisted initially by ligand binding simulations. Therefore, this study aimed to evaluate the binding potentials of various conventional drugs, vitamins, and plant-derived active compounds with SARS-CoV-2 main protease.
Materials and Methods
Protein preparation
The crystal structure of COVID-19 main protease (Mpro) with the ID 6LU7 [12] was retrieved as a PDB file from RCSB protein data bank and imported to Molegro Virtual Docker (MVD) [21]. Taking into consideration the fact that water molecules are not involved in the process of ligand-receptor binding; it is usually preferable to remove them before the molecular docking as this step can significantly enhance the computations and to avoid any probable distortion [22] Water molecules were then deleted and protein molecule was prepared prior to docking simulation via MVD molecule preparation function. Detect cavities function was then used in the search for proper docking constraints on the structure.
Ligands preparation
In total, 88 conventional antiviral drugs, 16 vitamins, and 63 natural (plant) compounds were chosen from the antivirus lists and literature [18-20][23-26]. All the chosen ligands were downloaded from ZINC15 database in SDF format and then imported into MVD workspace. The ligands were then prepared for docking using MVD molecule preparation function (Fig. 1).
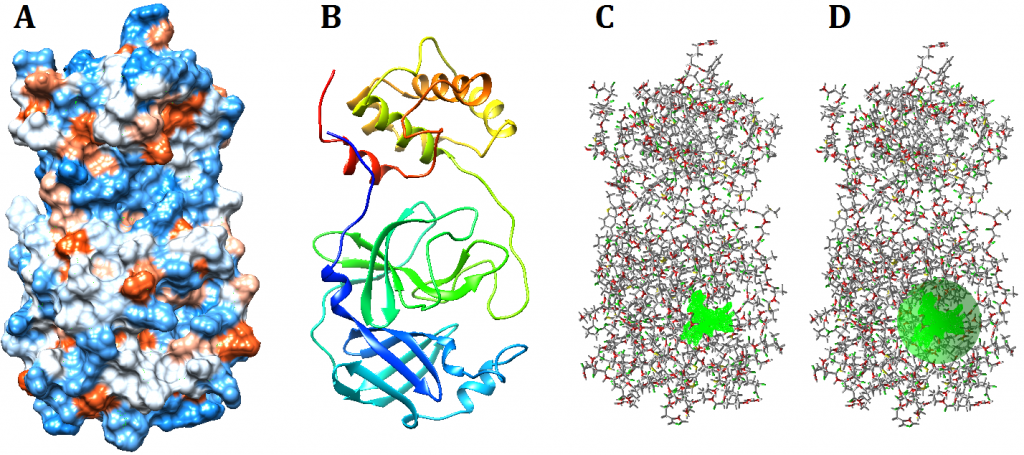
Docking and post-docking analysis
MVD docking wizard was started up and the protein structure along with all the chosen ligands were selected for docking. All docking specifications were left as the original default settings; however, binding site constrain was set to include the largest detected cavity on the protein structure and its dimensions were minimized in order to decrease the simulation processing time and increase accuracy (Fig. 1 D). Furthermore, the number of runs was set to 50 runs/ligand and the maximum population was set to 100 according to MVD recommendations as these settings resulted in better outcomes.
After completion, docking results were imported and the best pose of each ligand, based on their Re-rank score, was loaded into the workspace. The 2D diagrams of receptor-ligand interaction for the best poses were visualized using BIOVIA discovery space visualizer; while docking poses within the protein structure were visualized using UCSF Chimera software after exporting the protein molecule and poses in MOL2 format.
Results and Discussion
Cavity prediction function results demonstrated the presence of four cavities on the surface of Mpro which ranged in volume from 10.24 – 126.98 Å. The largest cavity was chosen for docking as it has originally included the biding ligand 02J-ALA-VAL-LEU-PJE-010 as provided in PDB (Fig. 1 C).
All the chosen compounds were evaluated for binding probability in the same docking session. After docking, the molecules were ranked based on their Re-rank score. The results showed that the best drugs were Tenofovir Alafenamide, Adefovir dipivoxil, Amprenavir, Raltegravir, Intelence, Ganciclovir, Ganciclovir Triphosphate, Pleconaril, Acyclovir Diphosphate, Doravirine, Rilpivirine, Valganciclovir, Victrelis, Stavudine, and Tenofovir Disoproxil with a re-rank scores ranged from -117.493 to -106.829 and MolDock scores between -167.369 and -125.673 (Table 1). Additionally, graphical visualization showed strong hydrogen bonds interaction with protein molecule (Fig. 2-6). All previously mentioned drugs are used in treatment programs of various viral diseases including HIV/AIDS, Cytomegalovirus (CMV), herpes, and Picornavirus (Table 1). Although some of these drugs have entered clinical trials of COVID-19, there are some reports of few with no significant positive results such as Ganciclovir and Acyclovir (no reports of Acyclovir Diphosphate) and therefore, these drugs were not recommended [1][44][45], additionally, Tenofovir Alafenamide trials [46] have also started with no new reports so far. The rest simulated drugs result can be seen in (Table 2).
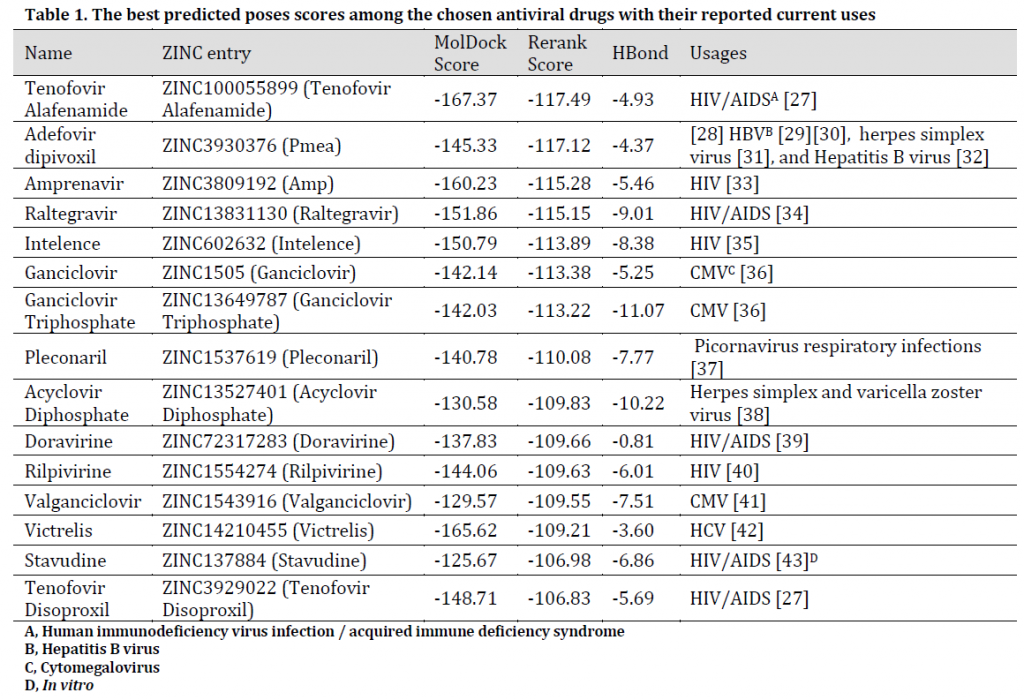
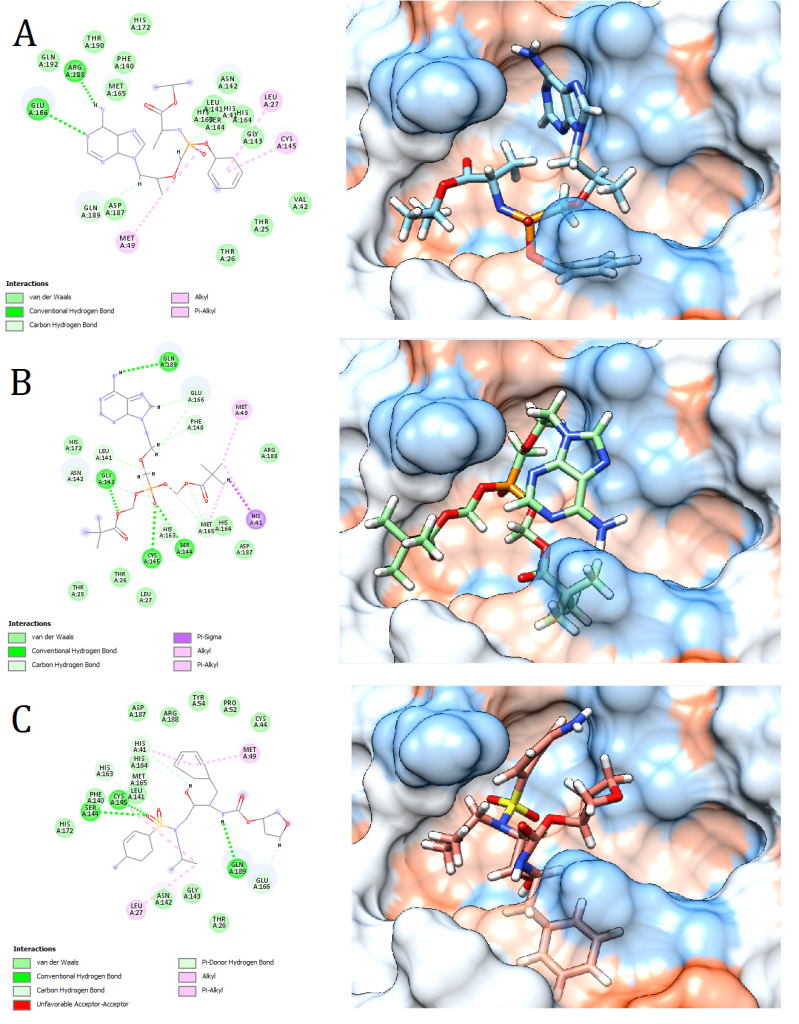
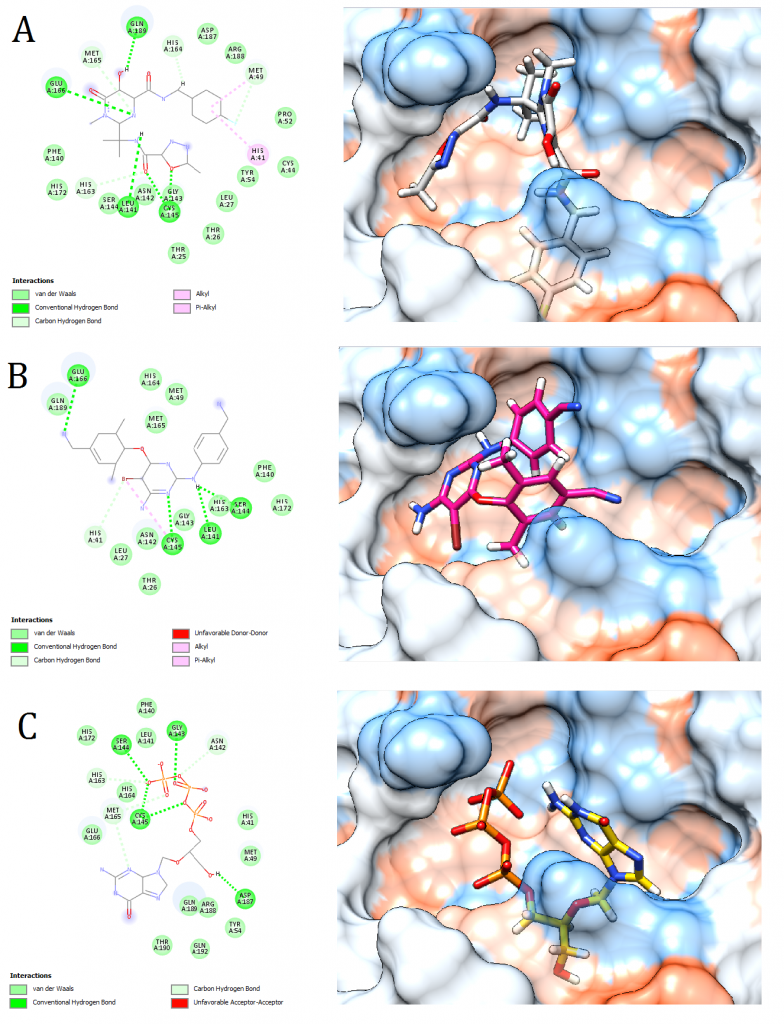
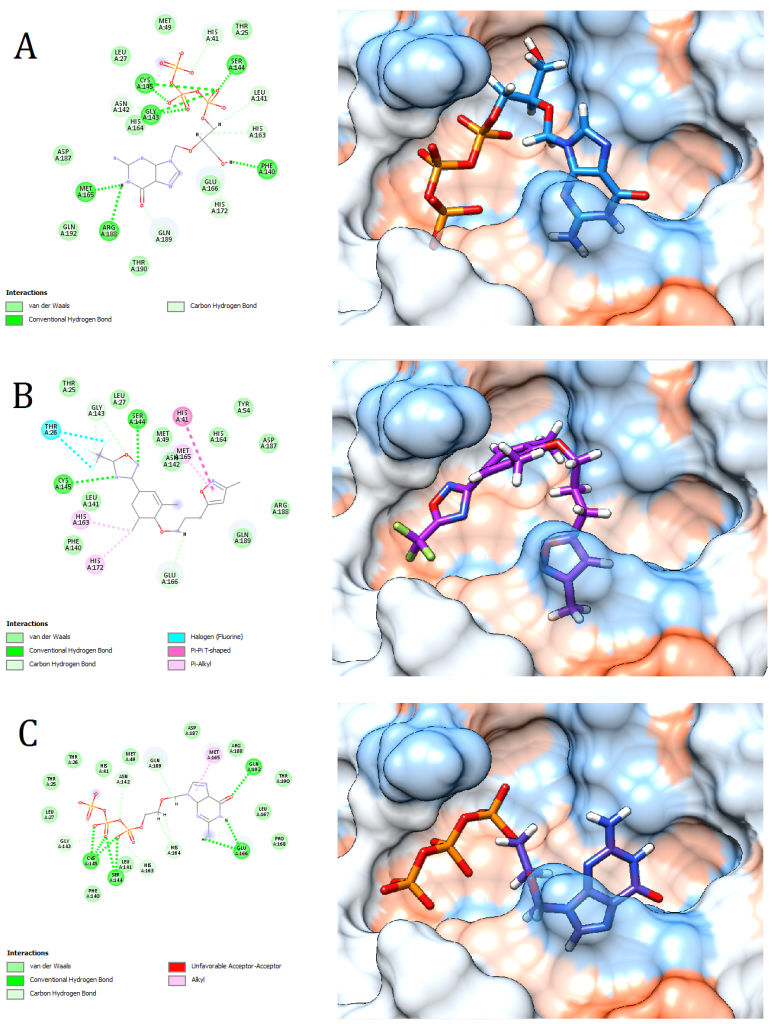
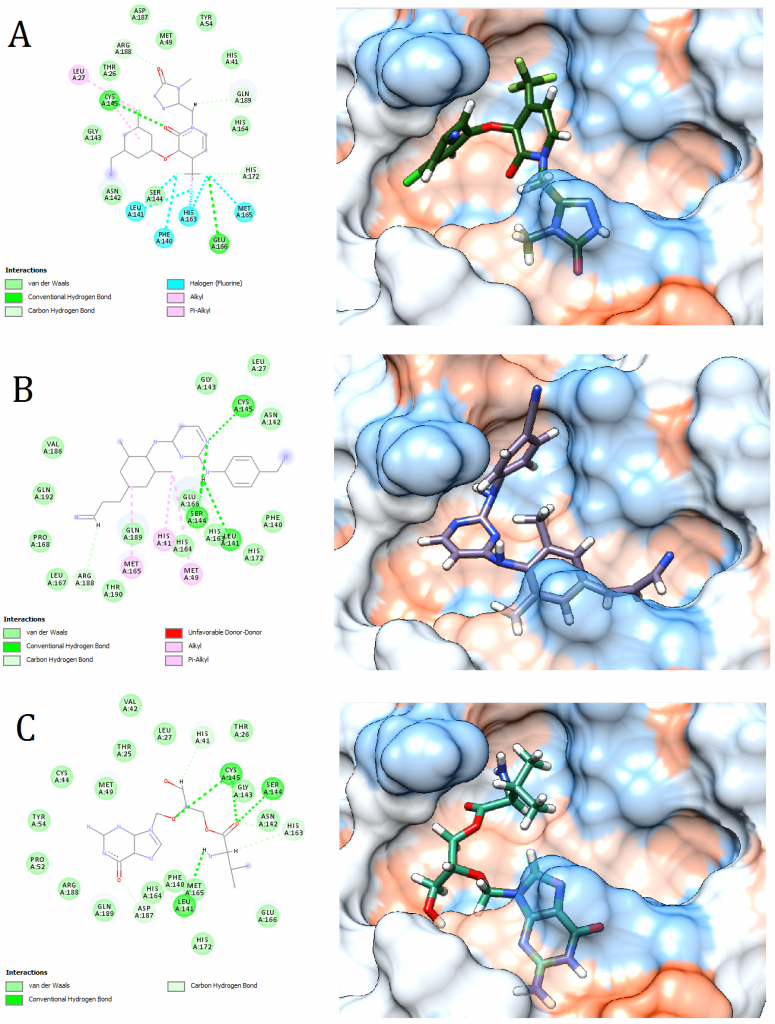
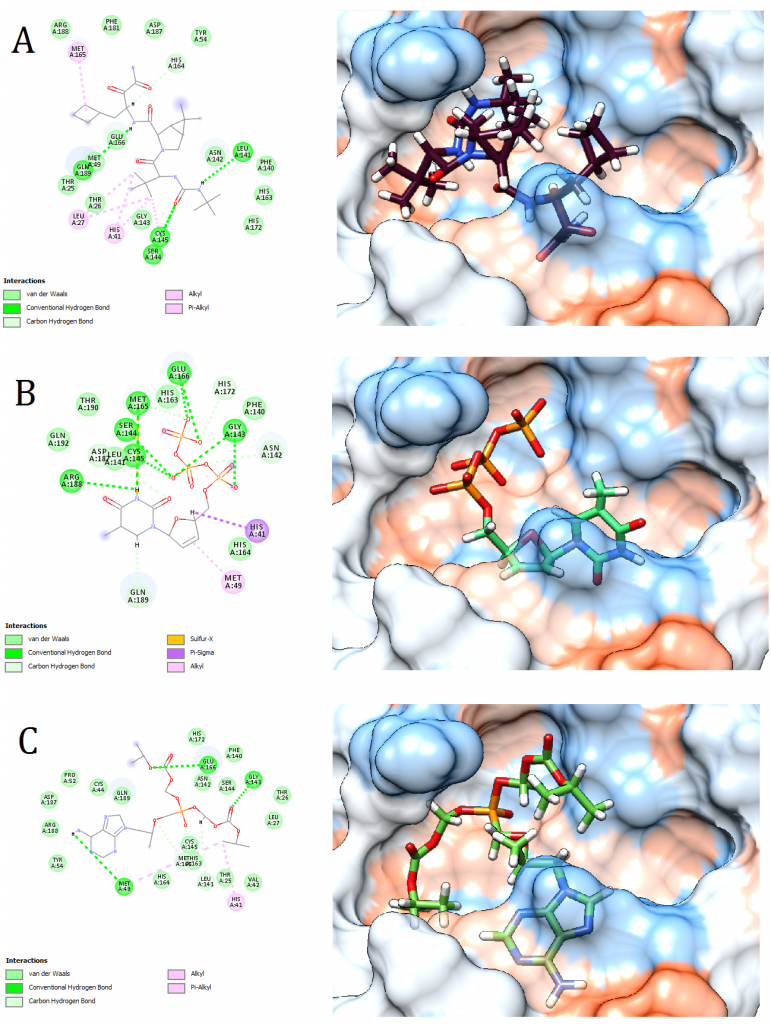
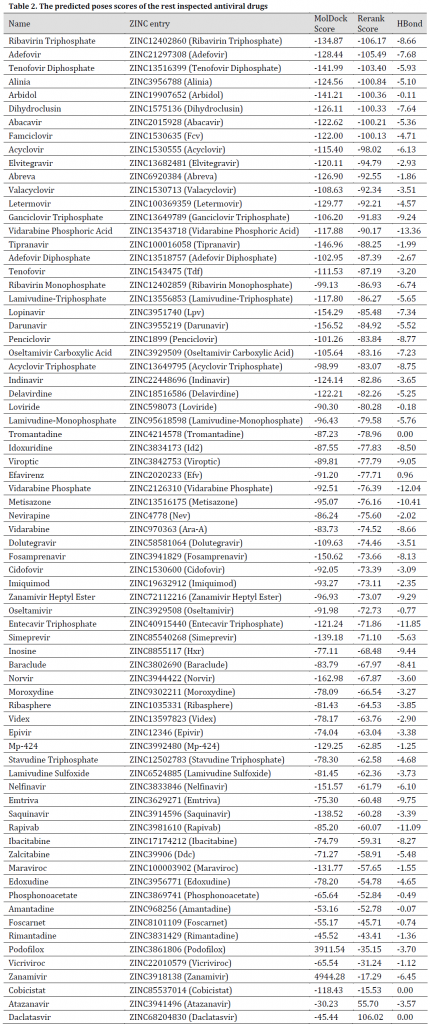
Among the investigated vitamins, B9, A, K, and E vitamins exhibited promising results with MolDock scores between -131.99 and -148.43 (Table 3). It is always recommended to fortify body immunity through a balanced diet that satisfies the daily body needs of vitamins and minerals. Vitamin B9 was additionally reported to have an Inhibitory effect on RNA-dependent-RNA polymerase activity of SARS-CoV-2 virus’s SCV2-nsp12 enzyme. On the other hand, Vitamin K3 was reported to have an inhibitory effect on HIV [47]. Additionally, vitamins E and D were reported to be proper candidates in improving body immunity towards COVID-19 [48], as low levels of these supplements increased viral infections with bovine coronavirus in cattle [49].
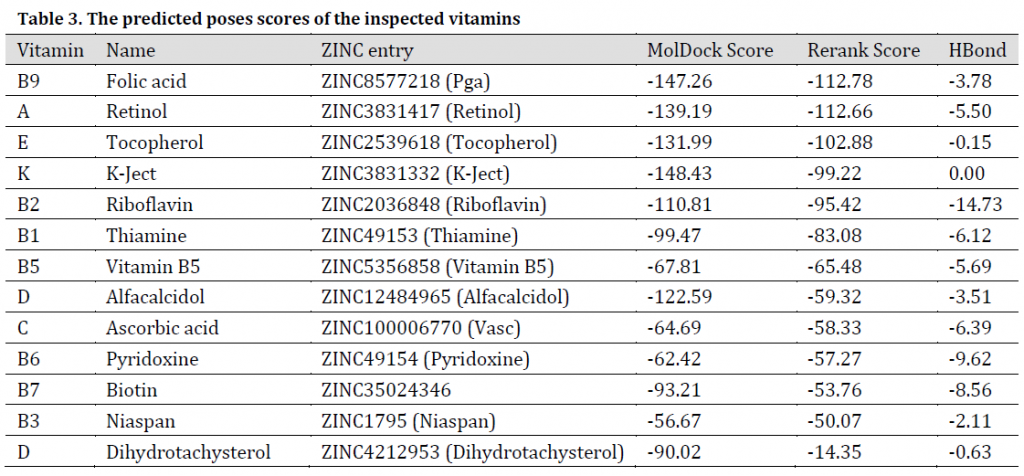
As for the studied plant natural compounds, five substances showed promising results. These substances were Curcumin (Curcuma longa), Mallotojaponin (Phyllanthus myrtifolius), Peltatol A (Pothomorphe peltata), Anolignan A (Anogeissus acuminata), and Phyllamyricin B (Phyllanthus myrtifolius) with rerank scores ranged between -131.59 and -107.42 with MolDock scores between -219.39 and -156.27 (Table 4) and various formed conventional Hydrogen bonds (Fig. 7 and 8). All these substances were reported as HIV inhibitors [24][50-54]. Curcumin was previously reported as a strong SARS main protease inhibitor [55]. The rest of the investigated natural-based molecules can be seen in (Table 5).
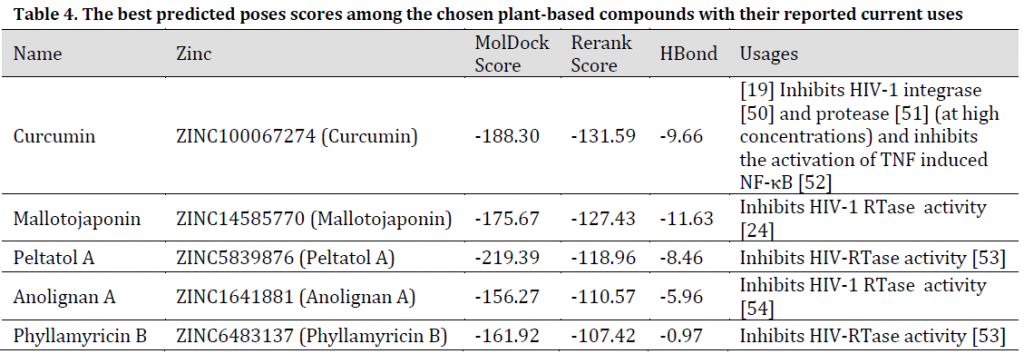
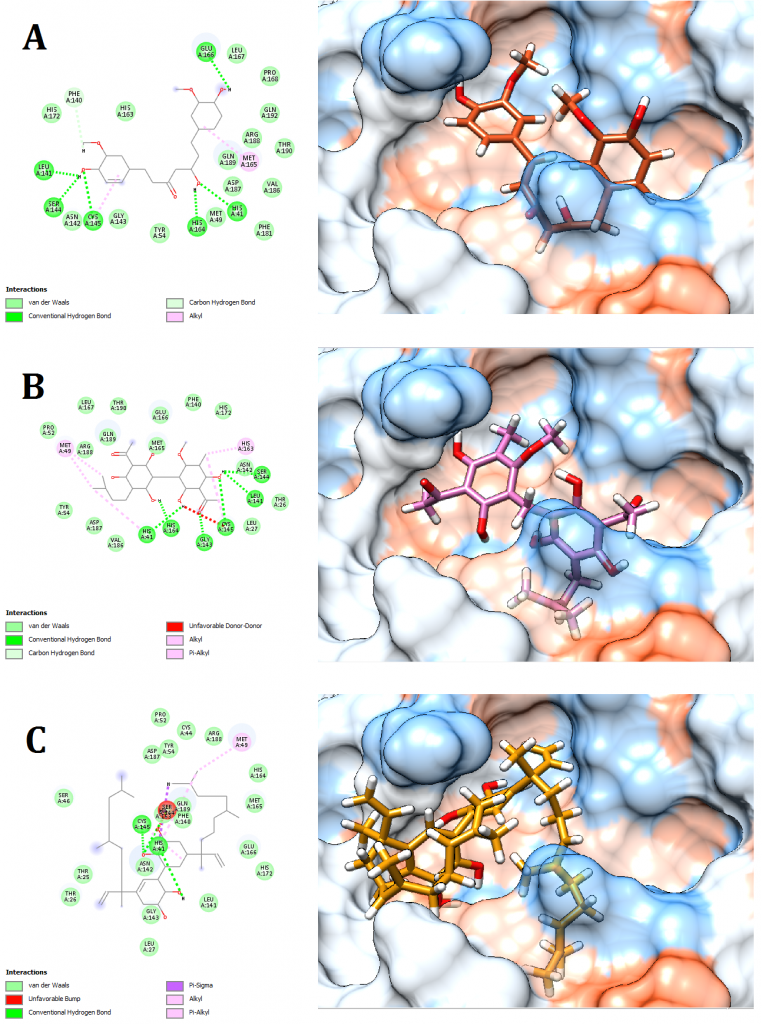
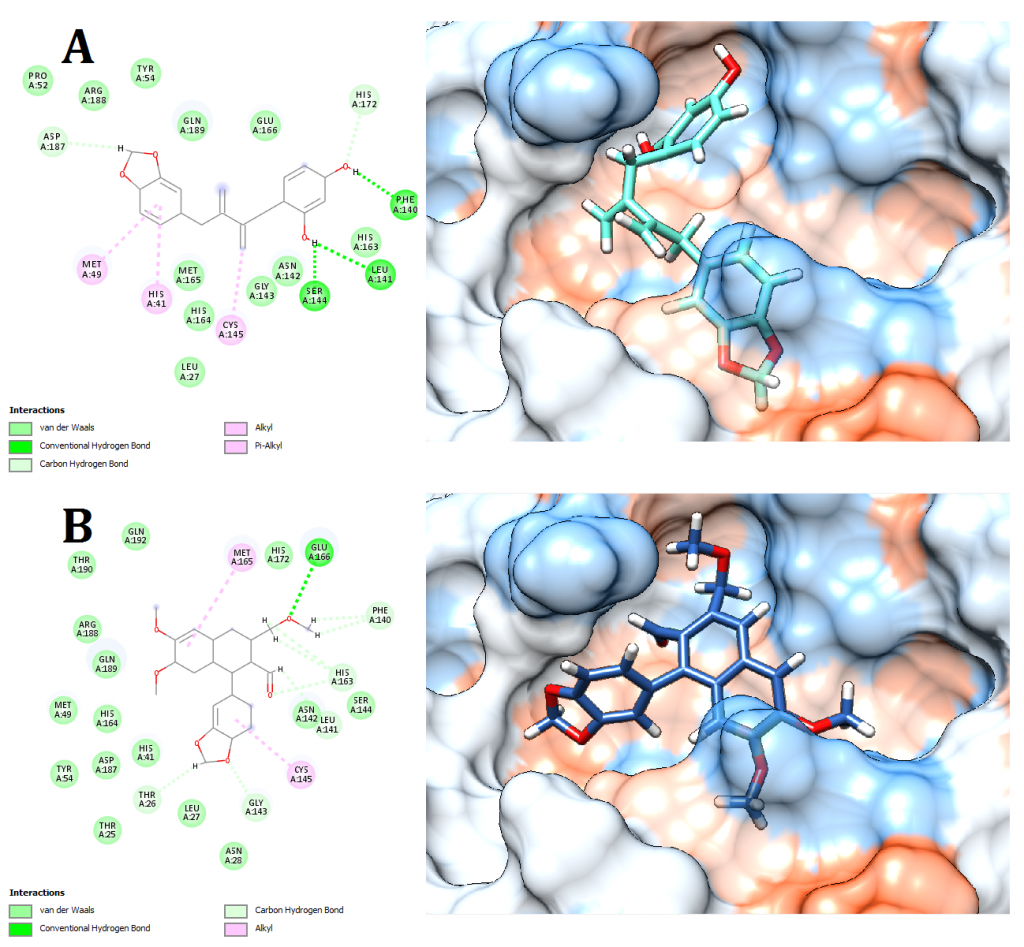
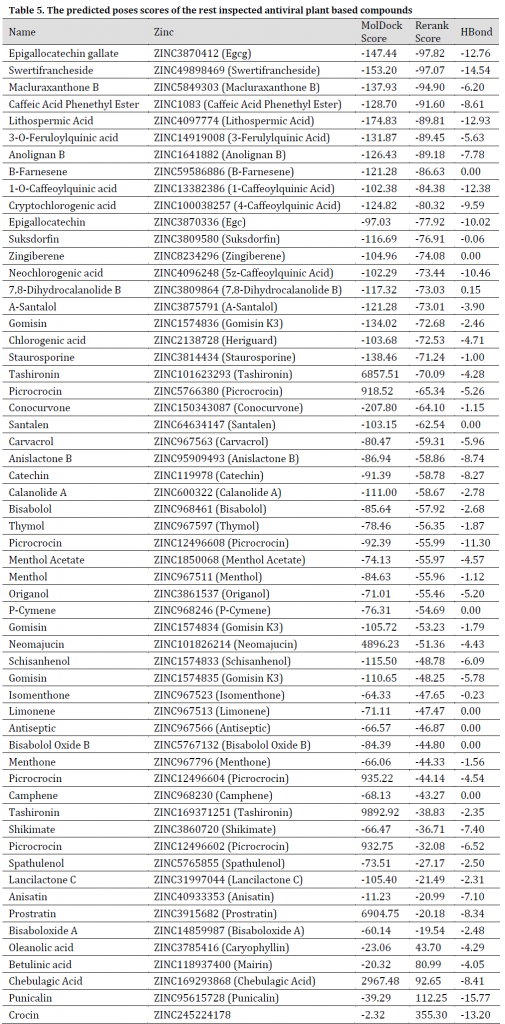
2D receptor-ligand interaction diagrams illustrated that among the 15 best drugs; Amprenavir, Intelence, Ganciclovir, Acyclovir Diphosphate, and Rilpivirine had an unfavorable acceptor-acceptor bond as can be seen in (Fig. 2 C), (Fig. 3 B), (Fig. 3 C), (Fig. 4 C), and (Fig. 5 B). On the other hand, among the 5 best natural products, Mallotojaponin had an unfavorable acceptor-acceptor bond (Fig. 7 B) while Peltatol A had an unfavorable bump (Fig. 7 C). Ganciclovir and Acyclovir were previously reported to have no inhibitory effect on the novel virus and were therefore not recommended for treatment which might be partially correlated to the predicted unfavorable bonds illustrated in the current study. However, in addition to their various conventional hydrogen bonds; the rest shortlisted drugs (except for Ganciclovir Triphosphate and Valganciclovir) and plant compounds were found to have various Alkyl and/or Pi-Alkyl bonds which might positively contribute to the hydrophobic ligand-receptor interaction [56]. Pi-Pi and fluorine bonding which can be seen in the interaction diagram of Pleconaril (Fig. 4 B) play an essential role in protein-ligand binding [57] and might render the binding of this drug as one of the strongest among the tested ligands [56]. Charge transfer which might be involved with Pi-sigma bonds present in Adefovir dipivoxil-protein and Stavudine-protein interaction diagrams (Fig. 2 B) and (Fig. 6 B) respectively can support an intercalating binding between these drugs and the receptor [56].
Conclusions
COVID-19 is without a doubt a crisis that grew to a global scale affecting the lives of billions worldwide. Therefore, all the efforts that may lead to a better understanding or provide significant relief of the major symptoms are highly welcomed. The results of the current study emphasize on the importance of vitamins in daily diets for their probable inhibitory effect on the virus especially B9, A, K, and E vitamins. Additionally, various conventional drugs and plant-based compounds have shown interesting ligand-protein interactions and therefore worth further investigation especially Pleconaril, Adefovir dipivoxil, and Stavudine in addition to Curcumin (Curcuma longa), Anolignan A (Anogeissus acuminata), and Phyllamyricin B (Phyllanthus myrtifolius). Molecular blockers for the virus or cell surface receptors were not investigated in the current study; however, these approaches might find other alternative potential drugs for this global epidemic.
References
1 | Guo YR, Cao QD, Hong ZS, Tan YY, Chen SD, Jin HJ, Tan KS, Wang DY, Yan Y. The origin, transmission and clinical therapies on coronavirus disease 2019 (COVID-19) outbreak–an update on the status. Mil. Med. Res. 2020;7(1):1-0. DOI |
2 | Zhou P, Yang XL, Wang XG, Hu B, Zhang L, Zhang W, Si HR, Zhu Y, Li B, Huang CL, Chen HD. A pneumonia outbreak associated with a new coronavirus of probable bat origin. Nature. 2020;579(7798):270-3. DOI |
3 | Li W, Shi Z, Yu M, Ren W, Smith C, Epstein JH, Wang H, Crameri G, Hu Z, Zhang H, Zhang J. Bats are natural reservoirs of SARS-like coronaviruses. Science. 2005;310(5748):676-9. DOI |
4 | Huang C, Wang Y, Li X, Ren L, Zhao J, Hu Y, et al. Clinical features of patients infected with 2019 novel coronavirus in Wuhan, China. Lancet. 2020;395(10223):497–506. DOI |
5 | Jin YH, Cai L, Cheng ZS, Cheng H, Deng T, Fan YP, Fang C, Huang D, Huang LQ, Huang Q, Han Y. A rapid advice guideline for the diagnosis and treatment of 2019 novel coronavirus (2019-nCoV) infected pneumonia (standard version). Mil. Med. Res. 2020 Dec 1;7(1):4. DOI |
6 | Lauer SA, Grantz KH, Bi Q, Jones FK, Zheng Q, Meredith HR, Azman AS, Reich NG, Lessler J. The incubation period of coronavirus disease 2019 (COVID-19) from publicly reported confirmed cases: estimation and application. Ann. Intern. Med. 2020 Mar 10. DOI |
7 | who.int [Internet]. WHO Director-General’s opening remarks at the media briefing on COVID-19 – 11 March 2020 [cited 2020 Mar 25]. Available from: Link |
8 | Hoffmann M, Kleine-Weber H, Schroeder S, Krüger N, Herrler T, Erichsen S, Schiergens TS, Herrler G, Wu NH, Nitsche A, Müller MA. SARS-CoV-2 cell entry depends on ACE2 and TMPRSS2 and is blocked by a clinically proven protease inhibitor. Cell. 2020. DOI |
9 | Adedeji AO, Severson W, Jonsson C, Singh K, Weiss SR, Sarafianos SG. Novel inhibitors of severe acute respiratory syndrome coronavirus entry that act by three distinct mechanisms. J. Virol. 2013;87(14):8017-28. DOI |
10 | Zhou Y, Hou Y, Shen J, Huang Y, Martin W, Cheng F. Network-based drug repurposing for novel coronavirus 2019-nCoV/SARS-CoV-2. Cell Discov. 2020;6(1):1-8. DOI |
11 | Xue X, Yu H, Yang H, Xue F, Wu Z, Shen W, Li J, Zhou Z, Ding Y, Zhao Q, Zhang XC. Structures of two coronavirus main proteases: implications for substrate binding and antiviral drug design. J. Virol. 2008;82(5):2515-27. DOI |
12 | Jin, Z., Du, X., Xu, Y. et al. Structure of Mpro from COVID-19 virus and discovery of its inhibitors. Nature (2020). DOI |
13 | Gao J, Tian Z, Yang X. Breakthrough: Chloroquine phosphate has shown apparent efficacy in treatment of COVID-19 associated pneumonia in clinical studies. Biosci. Trends. 2020. DOI |
14 | Touret F, de Lamballerie X. Of chloroquine and COVID-19. Antivir. Res. 2020:104762. DOI |
15 | Wang M, Cao R, Zhang L, Yang X, Liu J, Xu M, Shi Z, Hu Z, Zhong W, Xiao G. Remdesivir and chloroquine effectively inhibit the recently emerged novel coronavirus (2019-nCoV) in vitro. Cell Res. 2020;30(3):269-71. DOI |
16 | Gautret P, Lagier JC, Parola P, Meddeb L, Mailhe M, Doudier B, Courjon J, Giordanengo V, Vieira VE, Dupont HT, Honoré S. Hydroxychloroquine and azithromycin as a treatment of COVID-19: results of an open-label non-randomized clinical trial. Int. J. Antimicrob. Agents. 2020:105949. DOI |
17 | Chan KW, Wong VT, Tang SC. COVID-19: An Update on the Epidemiological, Clinical, Preventive and Therapeutic Evidence and Guidelines of Integrative Chinese–Western Medicine for the Management of 2019 Novel Coronavirus Disease. Am. J. Chin. Med. 2020:1-26. DOI |
18 | Heck CI, De Mejia EG. Yerba Mate Tea (Ilex paraguariensis): a comprehensive review on chemistry, health implications, and technological considerations. J. Food Sci. 2007;72(9):R138-51. DOI |
19 | Cos P, Maes L, Vanden Berghe D, Hermans N, Pieters L, Vlietinck A. Plant substances as anti-HIV agents selected according to their putative mechanism of action. J. Nat. Prod. 2004;67(2):284-93. DOI |
20 | Allahverdiyev AM, Bagirova M, Yaman S, Koc RC, Abamor ES, Ates SC, Baydar SY, Elcicek S, Oztel ON. Development of new antiherpetic drugs based on plant compounds. InFighting Multidrug Resistance with Herbal Extracts, Essential Oils and Their Components 2013:245-59. Academic Press. DOI |
21 | Thomsen R, Christensen MH. MolDock: a new technique for high-accuracy molecular docking. J. Med. Chem. 2006;49(11):3315-21. DOI |
22 | Wong SE, Lightstone FC. Accounting for water molecules in drug design. Expert opinion on drug discovery. 2011;6(1):65-74. |
23 | Wikipedia contributors. List of antiviral drugs [Internet]. Wikipedia, The Free Encyclopedia; 2020 Mar 20, 06:47 UTC [cited 2020 Mar 25]. Available from: Link |
24 | Patra JK, Das G, Bose S, Banerjee S, Vishnuprasad CN, del Pilar Rodriguez‐Torres M, Shin HS. Star anise (Illicium verum): Chemical compounds, antiviral properties, and clinical relevance. Phytother. Res. 2020. DOI |
25 | Kurapati KR, Atluri VS, Samikkannu T, Garcia G, Nair MP. Natural products as anti-HIV agents and role in HIV-associated neurocognitive disorders (HAND): a brief overview. Front. Microbiol. 2016;6:1444. DOI |
26 | Soleymani S, Zabihollahi R, Shahbazi S, Bolhassani A. Antiviral effects of saffron and its major ingredients. Curr. Drug Deliv. 2018;15(5):698-704. DOI |
27 | Sax PE, Wohl D, Yin MT, Post F, DeJesus E, Saag M, Pozniak A, Thompson M, Podzamczer D, Molina JM, Oka S. Tenofovir alafenamide versus tenofovir disoproxil fumarate, coformulated with elvitegravir, cobicistat, and emtricitabine, for initial treatment of HIV-1 infection: two randomised, double-blind, phase 3, non-inferiority trials. Lancet. 2015;385(9987):2606-15. DOI |
28 | Wikipedia contributors. Tenofovir alafenamide [Internet]. Wikipedia, The Free Encyclopedia; 2020 Mar 26, 05:58 UTC [cited 2020 Apr 2]. Available from: Link |
29 | Marcellin P, Chang TT, Lim SG, Tong MJ, Sievert W, Shiffman ML, Jeffers L, Goodman Z, Wulfsohn MS, Xiong S, Fry J. Adefovir dipivoxil for the treatment of hepatitis B e antigen–positive chronic hepatitis B. N. Engl. J. Med. 2003;348(9):808-16. DOI |
30 | Manolakopoulos S, Bethanis S, Koutsounas S, Goulis J, Vlachogiannakos J, Christias E, Saveriadis A, Pavlidis C, Triantos C, Christidou A, Papatheodoridis G. Long‐term therapy with adefovir dipivoxil in hepatitis B e antigen‐negative patients developing resistance to lamivudine. Alimentary pharmacology & therapeutics. 2008;27(3):266-73. DOI |
31 | ADHOC International Steering Committee. A randomized placebo‐controlled trial of adefovir dipivoxil in advanced HIV infection: the ADHOC trial. HIV Med. 2002;3(4):229-38. DOI |
32 | Qaqish RB, Mattes KA, Ritchie DJ. Adefovir dipivoxil: a new antiviral agent for the treatment of hepatitis B virus infection. Clin. Ther. 2003;25(12):3084-99. DOI |
33 | Noble S, Goa KL. Amprenavir. Drugs. 2000;60(6):1383-410. DOI |
34 | Steigbigel RT, Cooper DA, Kumar PN, Eron JE, Schechter M, Markowitz M, Loutfy MR, Lennox JL, Gatell JM, Rockstroh JK, Katlama C. Raltegravir with optimized background therapy for resistant HIV-1 infection. N. Engl. J. Med. 2008;359(4):339-54. |
35 | Eraikhuemen N, Thornton AM, Branch III E, Huynh ST, Farley C. Combating non-nucleoside reverse transcriptase inhibitor resistance with a focus on etravirine (Intelence) for HIV-1 infection. Pharm. Ther. 2008;33(8):445. |
36 | Martin DF, Kuppermann BD, Wolitz RA, Palestine AG, Li H, Robinson CA, Roche Ganciclovir Study Group. Oral ganciclovir for patients with cytomegalovirus retinitis treated with a ganciclovir implant. N. Engl. J. Med. 1999;340(14):1063-70. DOI |
37 | Hayden FG, Coats T, Kim K, Hassman HA, Blatter MM, Zhang B, Liu S. Oral pleconaril treatment of picornavirus-associated viral respiratory illness in adults: efficacy and tolerability in phase II clinical trials. Antivir. Ther. 2002;7(1):53-66. |
38 | Kawamura K, Hayakawa J, Akahoshi Y, Harada N, Nakano H, Kameda K, Ugai T, Wada H, Yamasaki R, Ishihara Y, Sakamoto K. Low-dose acyclovir prophylaxis for the prevention of herpes simplex virus and varicella zoster virus diseases after autologous hematopoietic stem cell transplantation. Int. J. Hematol. 2015;102(2):230-7. DOI |
39 | Colombier MA, Molina JM. Doravirine: a review. Curr Opin HIV AIDS. 2018;13(4):308-14. DOI |
40 | Jackson A, McGowan I. Long-acting rilpivirine for HIV prevention. Curr Opin HIV AIDS. 2015;10(4):253-7. DOI |
41 | Kimberlin DW, Jester PM, Sánchez PJ, Ahmed A, Arav-Boger R, Michaels MG, Ashouri N, Englund JA, Estrada B, Jacobs RF, Romero JR. Valganciclovir for symptomatic congenital cytomegalovirus disease. N. Engl. J. Med. 2015;372(10):933-43. DOI |
42 | Vermehren J, Park JS, Jacobson IM, Zeuzem S. Challenges and perspectives of direct antivirals for the treatment of hepatitis C virus infection. Int. J. Hepatol. 2018;69(5):1178-87. DOI |
43 | Nayak D, Boxi A, Ashe S, Thathapudi NC, Nayak B. Stavudine loaded gelatin liposomes for HIV therapy: Preparation, characterization and in vitro cytotoxic evaluation. Mater. Sci. Eng. C. 2017;73:406-16. DOI |
44 | Li H, Wang YM, Xu JY, Cao B. Potential antiviral therapeutics for 2019 Novel Coronavirus. Chinese journal of tuberculosis and respiratory diseases. 2020;43:E002-. DOI |
45 | Wang D, Hu B, Hu C, Zhu F, Liu X, Zhang J, Wang B, Xiang H, Cheng Z, Xiong Y, Zhao Y. Clinical characteristics of 138 hospitalized patients with 2019 novel coronavirus–infected pneumonia in Wuhan, China. Jama. 2020. DOI |
46 | Zhai P, Ding Y, Wu X, Long J, Zhong Y, Li Y. The epidemiology, diagnosis and treatment of COVID-19. Int. J. Antimicrob. Agents. 2020:105955. DOI |
47 | Min BS, Miyashiro H, Hattori M. Inhibitory effects of quinones on RNase H activity associated with HIV‐1 reverse transcriptase. Phytother. Res. 2002;16(S1):57-62. DOI |
48 | Wang LS, Wang YR, Ye DW, Liu QQ. A review of the 2019 Novel Coronavirus (COVID-19) based on current evidence. Int. J. Antimicrob. Agents. 2020:105948. DOI |
49 | Nonnecke BJ, McGill JL, Ridpath JF, Sacco RE, Lippolis JD, Reinhardt TA. Acute phase response elicited by experimental bovine diarrhea virus (BVDV) infection is associated with decreased vitamin D and E status of vitamin-replete preruminant calves. Journal of dairy science. 2014;97(9):5566-79. DOI |
50 | Sui Z, Salto R, Li J, Craik C, de Montellano PR. Inhibition of the HIV-1 and HIV-2 proteases by curcumin and curcumin boron complexes. Bioorg. Med. Chem. 1993;1(6):415-22. DOI |
51 | Singh S, Aggarwal BB. Activation of transcription factor NF-κB is suppressed by curcumin (diferuloylmethane). J. Biol. Chem. 1995;270(42):24995-5000. DOI |
52 | Natarajan K, Singh S, Burke TR, Grunberger D, Aggarwal BB. Caffeic acid phenethyl ester is a potent and specific inhibitor of activation of nuclear transcription factor NF-kappa B. Proc. Natl. Acad. Sci. U.S.A. 1996;93(17):9090-5. DOI |
53 | Liu JS, Li L. Schisantherins P and Q, two lignans from Kadsura coccinea. Phytochemistry. 1995;38(4):1009-11. DOI |
54 | Rimando AM, Pezzuto JM, Farnsworth NR, Santisuk T, Reutrakul V, Kawanishi K. New lignans from Anogeissus acuminata with HIV-1 reverse transcriptase inhibitory activity. J. Nat. Prod. 1994;57(7):896-904. DOI |
55 | Wen CC, Kuo YH, Jan JT, Liang PH, Wang SY, Liu HG, Lee CK, Chang ST, Kuo CJ, Lee SS, Hou CC. Specific plant terpenoids and lignoids possess potent antiviral activities against severe acute respiratory syndrome coronavirus. J. Med. Chem. 2007;50(17):4087-95. DOI |
56 | Arthur DE, Uzairu A. Molecular docking studies on the interaction of NCI anticancer analogues with human Phosphatidylinositol 4, 5-bisphosphate 3-kinase catalytic subunit. J. King Saud Univ. Sci. 2019;31(4):1151-66. DOI |
57 | Zhou P, Zou J, Tian F, Shang Z. Fluorine Bonding — How Does It Work In Protein− Ligand Interactions?. J Chem Inf Model. 2009;49(10):2344-55. DOI |
Cite this article:
Alabboud, M., Javadmanesh, A. In silico study of various antiviral drugs, vitamins, and natural substances as potential binding compounds with SARS-CoV-2 main protease. DYSONA – Life Science, 2020; 1(2): 44-63. doi: 10.30493/dls.2020.225404