Sahar M. Richi 1*
1, Geography Department, Faculty of Arts and Humanities, Tartous University, Tartous, Syria
E-mail:
saharrichi@tartous-univ.edu.sy
Received: 23/09/2024
Acceptance: 12/10/2024
Available Online: 12/10/2024
Published: 01/01/2025
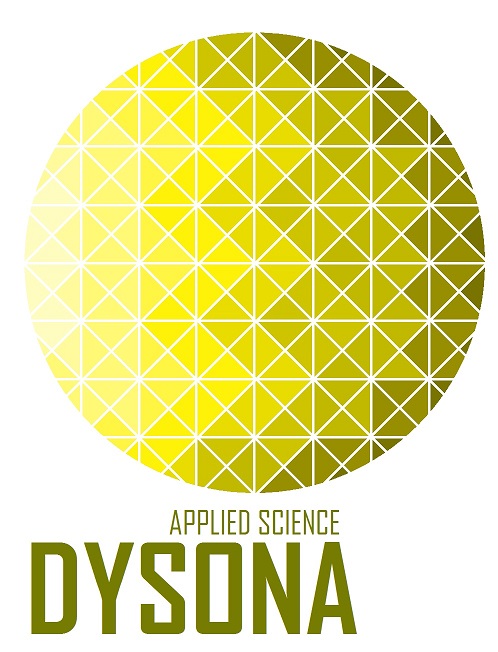
Manuscript link
http://dx.doi.org/10.30493/DAS.2024.479955
Abstract
Soil erosion is a critical risk facing the environmental and economic sustainability of agricultural land systems. It is considered among the most important problems that threaten ecosystems in the Syrian coastal region. This work aims to evaluate the spatial distribution of soil erosion risk in the Ghamima River Basin, especially in light of the lack of necessary data. For that purpose, the revised universal soil loss equation (RUSLE) model integrated with the geographic information system (GIS) and remote sensing (RS) data were used to formulate optimal soil erosion management plans and assess erosion risk levels. The results showed that the soil loss rate in the Ghamima River Basin ranges between 0 – 60 tons ha-1 year-1. The resulting soil erosion risk map was created and classified into five risk levels: very low (47.31%), low (28.38%), medium (12.61%), high (6.39%) and very high (5.31%). It was found that 11.7% of the lands of the study area are exposed to a high and very high degree of soil erosion risk. Accordingly, these results can be relied upon to support decision-makers in taking measures to mitigate the negative effects of soil erosion risk and designing soil protection strategies to prevent acceleration of erosion in high and very high-risk areas.
Keywords: Soil erosion, RUSLE, GIS, Syria
Introduction
Soil erosion is one of the most significant environmental threats, which may increase under the influence of climate change and the acceleration of human activities [1]. Soil erosion is a geophysical process that causes the destruction of the topsoil, thus losing nutrients [2], which primarily affects agricultural productivity [3]. At the river basin scale, accelerated soil erosion affects geophysical and geochemical processes in the topsoil, thus alters biodiversity and increases long-term maintenance costs [4].
Soil erosion results from a complex interaction between several factors including topography, soil properties, land cover, rainfall intensity, runoff volume, and agricultural practices [5]. Moreover, human activities have caused increased soil erosion through agricultural and urban expansions [6][7].
Soil erosion is considered one of the most prominent spatial challenges facing agricultural systems in the eastern Mediterranean in general and in Syria in particular [8]. In the western mountainous region of Syria, soil erosion rates are increasing due to several factors including, heavy rainfall, rugged terrain, sparse vegetation and accelerated human activity [9]. National literature indicates that about 18% of agricultural lands in Syria are at risk of soil loss exceeding 100 tons ha-1 year-1 in the western mountainous regions [10]. Therefore, spatial assessment of soil erosion risks is a fundamental approach to natural resource management and planning in river basins [11].
Numerous statistical models have been established for this purpose, including the Coordination of Information on the Environment (CORINE) [12] and the Water Erosion Prediction Project (WEPP) [13], and the Revised Universal Soil Loss Equation (RUSLE) [14-17], which are effective in quantifying the magnitude of the issue. These models have proven to be useful tools in assessing the risk of soil erosion in various environments, including the Mediterranean environment. In western Syria, CORINE, WEPP and RUSLE methods were used in spatial modeling of soil erosion [18-25].
A prominent instance of escalating soil erosion rates is the Ghamima River Basin in western Syria. However, there is no study to date that has evaluated erosion in this basin. Therefore, the aim of this research is to fill this gap through spatial assessment of soil erosion risk in the Ghamima River Basin, and to determine the degrees of soil erosion risk using the RUSLE model in a Geographic Information System (GIS) environment. The results of this assessment represent an important basis for decision-makers in improving the performance of soil erosion protection measures and implementing mitigation strategies.
Materials and Methods
Study area
The Ghamima River Basin is located in the western part of Syria – Tartous Governorate between Latitudes 34° 57ʹ – 34° 46ʹ North and Longitudes 36° 16ʹ – 35° 56ʹ East, with a total catchment area of 104.89 km2 (Fig. 1). The study area is bordered to the north by the Fawar River Basin and the Marqya River Basin, to the south by the Abrash River Basin and the Mentar River Basin, to the east by the Orontes (Al-Asi) River Basin, and to the west by the Lower Ghamqa River Basin and the Mediterranean. Geomorphologically, the study basin is divided into two main sectors: the first sector constitutes the plateau area (altitudes of 96 – 400 m) with an area ratio of 74% of the study area. The second sector constitutes the mountainous area (altitudes of 400 – 1119 m). This sector is characterized by steep slopes and slope instability, constituting 26% of the study area. Climatically, the study area belongs to the Mediterranean climate Csa according to the Köppen classification [26][27], which is characterized by rainy winters and hot, dry summers. The study basin has over 120 human communities, with land uses ranging from urban and agricultural to forested. Human activity patterns include agricultural, tourist, and service activities.
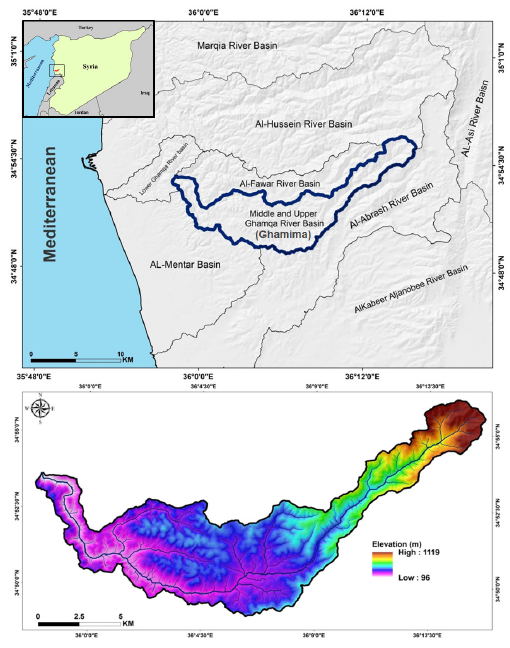
RUSLE model parameters
Scientists have developed several methods to estimate soil erosion through experimental, statistical and physical methods [28-31]. RUSLE is an empirical predictive model used to estimate average annual soil loss. This method evaluates the influence of climate, soil, topography and land use factors on soil erosion [32]. The RUSLE model consists of five geographic parameters including rainfall erosivity (R), soil erodibility (K), topography (LS), vegetation cover (C), and erosion control practices (P). The average annual soil erosion per unit area is given according to Eq.1 (Table 1) [31], where A is the average annual soil erosion (tons ha-1 year-1), R is the rainfall erosivity, K is the soil erodibility, LS is the hill slope length and steepness, C is the vegetation factor, and P is the support practice.
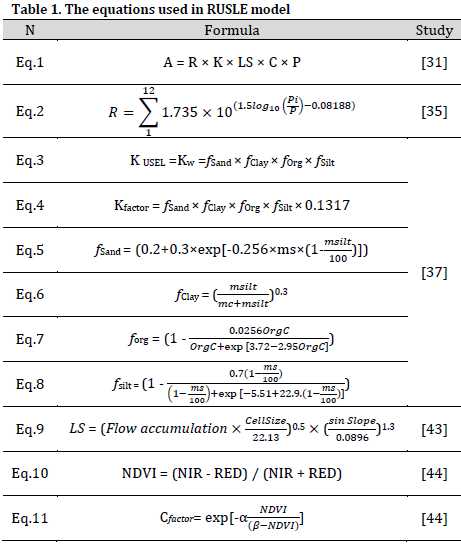
In this evaluation, the RUSLE equation was used along with geospatial techniques to analyze soil erosion by producing maps of all the main influencing factors. However, the RUSLE model was chosen based on its high quality in providing reliable outputs at the level of river basins, especially in the Eastern Mediterranean region [10][24].
Rainfall erosivity factor (R)
The literature indicates that erosion rates will increase due to the positive effect of global climate change on the intensity of rainstorms [33]. R factor indicates the ability of rainfall to cause erosion. Rainfall intensity, kinetic energy, and droplet size are the main factors that induce soil loss during rainstorms [34]. The original method for calculating
the values of R factor for a rainfall event requires rainfall records at 30-minute time steps. The calculation of R values using the previous method can only be applied in areas equipped with measuring stations that record rainfall in real time. According to the available monthly rainfall data of 4 climate stations established in and around the study basin. Precipitation data was obtained from the General Directorate of Meteorology in Damascus Governorate, Syria. R value map was prepared using Eq.2 [35] (Table 1), where R is a rainfall erosivity factor (MJ mm ha–1 h–1 year-1); Pi is monthly rainfall (mm); P is an annual rainfall (mm). R factor values ranged from 889.422 to 1479.69 MJ mm ha–1 h–1 year-1 (Fig. 2 A).
Erodibility factor (K)
The erodibility factor (K) represents the effect of various soil properties including organic matter content, soil structure, and particle size on the erodibility of slopes (K) [31]. K factor is a key factor when estimating soil loss. The K factor is simply defined as the innate erodibility of soil [36]. Soil data were obtained from the Global Soil Grid Database (From Link). To calculate the K factor, the data were processed according to the equations of Williams (1995) [37] Eq.3-8 (Table 1), which have been used by many soil erosion researchers [23][38-40]. K represents the erodibility factor, while ms, msilt, mc, and OrgC represent the percentage of sand, silt, clay, and organic matter, respectively. The spatial distribution of K values in study area ranged from 0.051 to 0.055 t ha-1 MJ-1 mm-1 (Fig. 2 B).
Slope length and steepness factor (LS)
To evaluate the effect of topography on soil erosion, the concept of the unit-contributing area was used. This method is effective in areas with complex topography [41], as it takes into account the contributing area and runoff accumulation [42]. The RUSLE method for calculating the LS factor uses slope length, angle, and a factor based on the slope steepness as a percentage [43]. Digital elevation model (DEM) was obtained from the Alaska Satellite Facility (From Link) and the LS map was extracted from the digital elevation model using Eq.9 (Table 1).
FlowAccumulation is the grid layer for flow accumulation expressed as the number of grid cells, and CellSize is the length of the side of the cell. The slope values ranged from 0 to 46.4 degrees and LS values ranged from 0 to 23.925 (Fig. 2 C and D, respectively).
Vegetation factor (C)
The factor of vegetation cover, agricultural systems, and land use (C) influences runoff and erosion rates and is estimated based on satellite imagery obtained from Sentinel-2 satellite (August 2023). First, Eq.10 [44] (Table 1) was used to estimate Normalized Difference Vegetation Index (NDVI), where NIR is the near infrared band (band 8, located in the spectral range 0.665 mµ), and RED is the red band (band 4, 0.842 mµ). NDVI values ranged from -0.068 to 0.63 (Fig. 2 E). The C-factor was then calculated according to Eq.11 (Table 1), where the parameters α and β determine the shape of the vegetation index curve. Reasonable results are obtained with values of α = 2 and β = 1 ranging from 0.035 to 1 (Fig. 2 F).
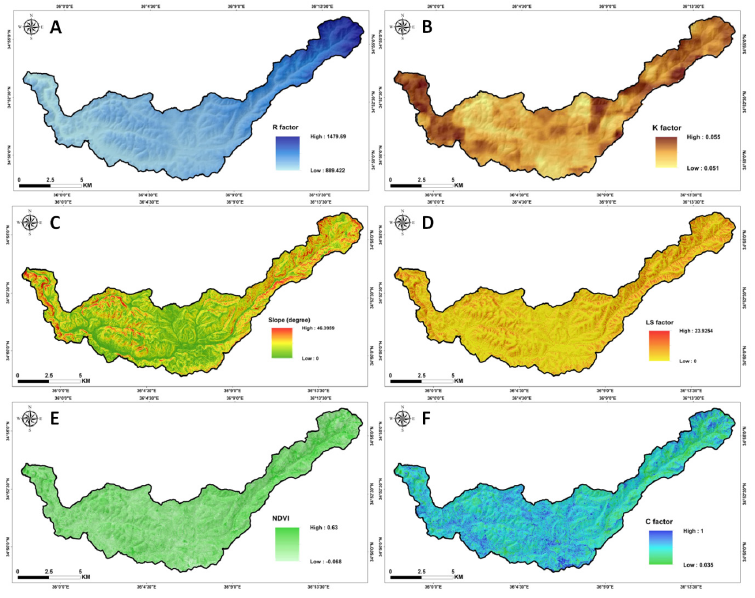
Conservation factor (P)
The P factor represents conservation and maintenance practices such as contour farming, strip farming, and terraces that reduce the rate of water erosion in a specific location [45]. Conservation and maintenance practices can negatively affect water erosion by modifying the flow pattern (drainage patterns), slope, or direction of runoff and reducing the volume and velocity of runoff [31]. The more effective the practice method, the lower the p value. The field work carried out revealed the poor or absence of conservation and maintenance measures in the study area. Consequently, the value of 1 was given to the P factor in the entire studied area.
Results and Discussion
Based on the initial inputs in the GIS environment, maps of the factors involved in the RUSLE equation were created. Using the raster calculator tool in the GIS environment, the raster parameters of R, K, LS and C were multiplied spatially in order to produce a spatial distribution map of potential soil loss per hectare per year at the cell level (Fig. 3). The results showed that the annual rate of potential soil loss was 60 tons ha-1 year-1 with a spatial average of 1-ton ha-1 year-1. The soil erosion risk map was classified using the natural dividers method into 5 categories, including very low (47.31%), low (28.38%), medium (12.61%), high (6.39%) and very high (5.31%) as shown (Table 2). The high and very high potential erosion risk values were dispersed over several regions of the basin, particularly in the northeastern mountainous area where slope gradients attain up to 46.3 degrees, hence exacerbating erosion.
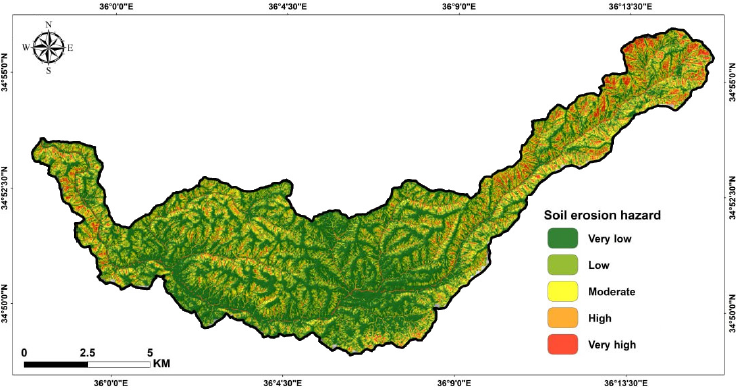
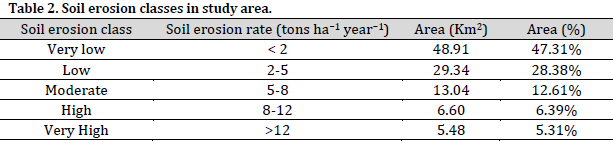
The current results are consistent with the literature on soil erosion assessment conducted in the Mediterranean environment [8][9][23][46-48]. Soil loss tolerance serves as a valuable criterion for evaluating the potential for production decline and associated economic repercussions, as well as for formulating soil erosion control measures and implementing soil conservation activities [49]. Mediterranean studies indicate that the erosion tolerance threshold that ensures agricultural and economic sustainability ranges from 2 to 12 tons ha-1 year-1 [48][50-52]. As for the Syrian coastal region, the acceptable limit for soil erosion ranges from 1 to 2.5 tons ha-1 year-1 [53][54]. Therefore, the research region requires immediate and comprehensive spatial management of soil erosion, encompassing the development of conservation and mitigation techniques. The RUSLE model yielded valuable insights for decision-makers to formulate enhanced sustainable management strategies that safeguard soils in regions of high and very high erosion susceptibility.
Conclusion
Soil erosion is a significant geomorphological hazard impacting spatial development in the Syrian coastal region, adversely affecting agricultural and economic productivity, and therefore, food security. This work employed spatial methodologies, including geographic information systems, utilizing multi-source data, particularly remote sensing data, to generate RUSLE model factor maps. This study evaluated the geographical distribution of soil erosion susceptibility, indicating a possible annual soil loss rate of 60 tons ha-1 year-1. 11.7% of the studied region exhibited high and very high erosion susceptibility. Consequently, the findings of this study are crucial for managing soil erosion risks through the implementation of suitable mitigation and conservation strategies.
References
- Bezak N, Borrelli P, Mikoš M, Jemec Auflič M, Panagos P. Towards multi-model soil erosion modelling: An evaluation of the erosion potential method (EPM) for global soil erosion assessments. CATENA. 2024;234:107596. DOI
- Li P, Tariq A, Li Q, Ghaffar B, Farhan M, Jamil A, Soufan W, El Sabagh A, Freeshah M. Soil erosion assessment by RUSLE model using remote sensing and GIS in an arid zone. Int. J. Digit. Earth. 2023;16(1):3105-24. DOI
- Polykretis C, Grillakis MG, Manoudakis S, Seiradakis KD, Alexakis DD. Spatial variability of water-induced soil erosion under climate change and land use/cover dynamics: From assessing the past to foreseeing the future in the Mediterranean island of Crete. Geomorphology. 2023;439:108859. DOI
- Ahmad WS, Jamal S, Taqi M, El-Hamid HTA, Norboo J. Estimation of soil erosion and sediment yield concentrations in Dudhganga watershed of Kashmir Valley using RUSLE and SDR model. Environ. Dev. Sustain. 2022;26(1):215–38. DOI
- Islam MdR, Imran HM, Islam MdR, Saha GC. A RUSLE-based comprehensive strategy to assess soil erosion in a riverine country, Bangladesh. Environ. Earth Sci. 2024;83(6). DOI
- Li Y, Ni J, Yang Q, Li R. Human Impacts on Soil Erosion Identified Using Land-Use Changes: A Case Study From The Loess Plateau, China. Phys. Geogr. 2006;27(2):109–26. DOI
- Rapuc W, Giguet-Covex C, Bouchez J, Sabatier P, Gaillardet J, Jacq K, Genuite K, Poulenard J, Messager E, Arnaud F. Human-triggered magnification of erosion rates in European Alps since the Bronze Age. Nat. Commun. 2024;15(1):1246. DOI
- Alsafadi K, Bi S, Abdo HG, Al Sayah MJ, Ratonyi T, Harsanyi E, Mohammed S. Spatial–temporal dynamic impact of changes in rainfall erosivity and vegetation coverage on soil erosion in the Eastern Mediterranean. Environ. Sci. Pollut. Res. 2024;31(28):40511-29. DOI
- Abdo HG. Evaluating the potential soil erosion rate based on RUSLE model, GIS, and RS in Khawabi river basin, Tartous, Syria. DYSONA Appl. Sci. 2022;3(1):24-32. DOI
- Abdo HG. Estimating water erosion using RUSLE, GIS and remote sensing in Wadi-Qandeel river basin, Lattakia, Syria. Proc. Indian Natl. Sci. Acad. 2021;87(3):514–23. DOI
- Ansari A, Tayfur G, Mohammadi S. Assessment of soil erosion and sediment delivery ratio in the Arghandab Catchment, Kandahar Province, Afghanistan by using GIS-based RUSLE method. Geomatics Nat. Hazards Risk. 2024;15(1). DOI
- Nur AH, Ahmed AH, Mohamed AA, Hasan MF, Sarmin S. Geospatial Assessment of Aridity and Erosivity Indices in Northwest Somalia Using the CORINE Model. J. Environ. Sci. Educ. 2024;4(1). DOI
- Albaradeyia I, Hani A, Shahrour I. WEPP and ANN models for simulating soil loss and runoff in a semi-arid Mediterranean region. Environ. Monit. Assess. 2010;180(1–4):537–56. DOI
- Weslati O, Serbaji MM. Spatial Assessment of Soil Erosion Using RUSLE Model, Remote Sensing and GIS: A Case Study of Mellegue Watershed, Algeria- Tunisia. Environ. Monit. Assess. 2023; DOI
- Hamouch C, Chaaouan J, eddine Bouiss C. Spatial-temporal assessment of soil erosion using the RUSLE model in the upstream Inaouène watershed, Northern Morocco. Nat. Hazards Res. 2024. DOI
- Erdoğan Yüksel E, Karan ÖF, Akay AE. Spatio-Temporal Analysis of Erosion Risk Assessment Using GIS-Based AHP Method: A Case Study of Doğancı Dam Watershed in Bursa (Türkiye). Forests. 2024;15(7):1135. DOI
- Sifi S, Aydi A, Bouamrane A, Zaghdoudi S, Gasmi M. Appraisal of soil erosion risk in northeastern Tunisia using geospatial data and integrated approach of RUSLE model and GIS. J. Earth Syst. Sci. 2024;133(2). DOI
- Barakat M, Mahfoud I, Kwyes AA. Study of soil erosion risk in the basin of Northern Al-Kabeer river at Lattakia-Syria using remote sensing and GIS techniques. Mesopotam. J. Mar. Sci. 2022;29(1):29–44. DOI
- Husein HH, Kalkha M. Soil erosion risk in the rainy mountainous area of eastern Mediterranean. In Global Symposium on Soil Erosion. 2019:260.
- Al-Rubaye A, Barakat M, Mahmood AB. Spatial distribution of soil water erosion at Al-Hawiz Dam in Western Syria using CORINE model. Mesopotam. J. Mar. Sci. 2021;35(1):1–12. DOI
- Mohammed S, Kbibo I, Alshihabi O, Mahfoud E. Studying rainfall changes and water erosion of soil by using the WEPP model in Lattakia, Syria. J. Agric. Sci. Belgrade. 2016;61(4):375–86. DOI
- Gassar AA, Aishihabi O. Studying Runoff and Water Erosion by Using Geowepp Model in Sheikh Basr Region-Syria. Ecol. Environ. Conserv. 2014.
- Al-hasn R, Alghamaz F, Dikkeh M, Idriss Y. Water soil erosion modeling with RUSLE, GIS & remote sensing: A case study of the AL-Sanawbar River basin (Syria). J. Saudi Soc. Agric. Sci. 2024. DOI
- Abdo HG, Almohamad H, Al Dughairi AA, Al-Mutiry M. Quantifying the water soil erosion rate using RUSLE, GIS, and RS approach for Al-Qshish River Basin, Lattakia, Syria. Geofizika. 2022;39(2):223–41. DOI
- Mohammed S, Hussien M, Alsafadi K, Mokhtar A, Rianna G, Kbibo I, Barkat M, Talukdar S, Szabó S, Harsanyi E. Assessing the WEPP model performance for predicting daily runoff in three terrestrial ecosystems in western Syria. Heliyon. 2021;7(4). DOI
- Mohammed S, Al-Ebraheem A, Holb IJ, Alsafadi K, Dikkeh M, Pham QB, Linh NT, Szabo S. Soil management effects on soil water erosion and runoff in central Syria—A comparative evaluation of general linear model and random forest regression. Water. 2020;12(9):2529. DOI
- Beck HE, Zimmermann NE, McVicar TR, Vergopolan N, Berg A, Wood EF. Present and future Köppen-Geiger climate classification maps at 1-km resolution. Sci. Data. 2018;5(1). DOI
- Fredj A, Ghernaout R, Dahmani S, Remini B. Assessing soil erosion through the implementation of the RUSLE model and geospatial technology in the Isser watershed, northern Algeria. Water Supply. 2024;24(7):2487–505. DOI
- Wischmeier WH. Predicting rainfall-erosion losses from cropland east of the Rocky Mountains, Guide for selection of practices for soil and water conservation. United States Government Printing Office. 1965.
- Williams JR. Sediment-yield prediction with universal equation using runoff energy factor¹. In Present and Prospective Technology for Predicting Sediment Yield and Sources: Proceedings of the Sediment-Yield Workshop, USDA Sedimentation Laboratory, Oxford, Miss. Agricultural Research Service, US Department of Agriculture. 1975;40:244.
- Renard KG. Predicting soil erosion by water: a guide to conservation planning with the Revised Universal Soil Loss Equation (RUSLE). US Department of Agriculture, Agricultural Research Service. 1997.
- Mete B, Bayram A. Effects of sediment-storage dams on suspended sediment transport in the Sera Lake Watershed, northeast Turkey. Environ. Earth Sci. 2024;83(8). DOI
- González-Hidalgo JC, Peña-Monné JL, de Luis M. A review of daily soil erosion in Western Mediterranean areas. CATENA. 2007;71(2):193–9. DOI
- Fellah S, Benzater B, Guemou L, Hachemaoui A, Benzohra MN, Elouissi A, Hamimed A. Using USLE, GIS and remote sensing for the soil loss assessment in the National Park of Theniet El Had, Algeria. J. Agric. Appl. Biol. 2024;5(2):178-93.
- Wischmeier WH, Smith DD. Predicting rainfall erosion losses: a guide to conservation planning. Department of Agriculture, Science and Education Administration. 1978.
- Luvai A, Obiero J, Omuto C. Soil Loss Assessment Using the Revised Universal Soil Loss Equation (RUSLE) Model. Appl. Environ. Soil Sci. 2022;2022:1–14. DOI
- Williams JR, Singh V. The EPIC model. Computer models of watershed hydrology. Water Resour. Publ. Highl. Ranch Colo. 1995;25:909-1000.
- Pakoksung K. Assessment of Soil Loss from Land Cover Changes in the Nan River Basin, Thailand. GeoHazards. 2024;5(1):1–21. DOI
- Djoukbala O, Djerbouai S, Alqadhi S, Hasbaia M, Benselama O, Abdo HG, Mallick J. A geospatial approach-based assessment of soil erosion impacts on the dams silting in the semi-arid region. Geomatics Nat. Hazards Risk. 2024;15(1):2375543. DOI
- Sarwar A, Ali M, Israr M, Gulzar S, Khan MI, Ali MA, Majid A, Rukh S. Mapping annual soil loss in the southeast of Peshawar basin, Pakistan, using RUSLE model with geospatial approach. Geol. Ecol. Landsc. 2024:1-2. DOI
- Shojaeezadeh SA, Al-Wardy M, Nikoo MR, Mooselu MG, Talebbeydokhti N, Alamdari N, Gandomi AH. Historical Hazard Assessment of Climate and Land Use–Land Cover Effects on Soil Erosion Using Remote Sensing: Case Study of Oman. Remote Sens. 2024;16(16):2976. DOI
- Desmet PJ, Govers G. A GIS procedure for automatically calculating the USLE LS factor on topographically complex landscape units. J. Soil Water Conserv. 1996;51(5):427-33.
- Benavidez R, Jackson B, Maxwell D, Norton K. A review of the (Revised) Universal Soil Loss Equation ((R)USLE): with a view to increasing its global applicability and improving soil loss estimates. Hydrol. Earth Syst. Sci. 2018;22(11):6059–86. DOI
- Okenmuo FC, Ewemoje TA. Estimation of soil water erosion using RUSLE, GIS, and Remote Sensing in Obibia River watershed, Anambra, Nigeria. DYSONA Appl. Sci. 2023;4(1):6-14. DOI
- Panagos P, Borrelli P, Meusburger K, van der Zanden EH, Poesen J, Alewell C. Modelling the effect of support practices (P-factor) on the reduction of soil erosion by water at European scale. Environ. Sci. Policy. 2015;51:23–34. DOI
- Benamor S, Messaid B, Berghout A. Estimation and mapping of soil erosion using the RUSLE model and GIS tools: A case study of the wad El Hai watershed in the western Aurès, north-eastern Algeria. Bull. Serb. Geogr. Soc. 2024;104(1):55–72. DOI
- Almohamad H. Impact of Land Cover Change Due to Armed Conflicts on Soil Erosion in the Basin of the Northern Al-Kabeer River in Syria Using the RUSLE Model. Water. 2020;12(12):3323. DOI
- Farhan Y, Nawaiseh S. Spatial assessment of soil erosion risk using RUSLE and GIS techniques. Environ. Earth Sci. 2015;74(6):4649–69. DOI
- Di Stefano C, Nicosia A, Pampalone V, Ferro V. Soil loss tolerance in the context of the European Green Deal. Heliyon. 2023;9(1):e12869. DOI
- Nearing MA, Deer-Ascough L, Laflen JM. Sensitivity analysis of the WEPP hillslope profile erosion model. Trans. ASAE. 1990;33(3):839-0849. DOI
- İrvem A, Topaloğlu F, Uygur V. Estimating spatial distribution of soil loss over Seyhan River Basin in Turkey. J. Hydrol. 2007;336(1–2):30–7. DOI
- Trabucchi M, Puente C, Comin FA, Olague G, Smith SV. Mapping erosion risk at the basin scale in a Mediterranean environment with opencast coal mines to target restoration actions. Reg. Environ. Change. 2012;12(4):675–87. DOI
- Kbibo I, Nesafi I. Water erosion and impacts on the coastal area in the Syrian Arab Republic. Tishreen Univ. J. Stud. Sci. Res. 1997;18:59-76.
- Ibrahiem N. Soil water erosion and soil conservation in Syrian Arab Republic. Univ. Aleppo J. (In Arabic) 1986;6(1):79–110.
Cite this article:
Richi, S. Integrated RUSLE-GIS modeling for enhancing soil erosion management in Ghamima River Basin, Syria. DYSONA – Applied Science, 2024;6(1): 104-112. doi: 10.30493/das.2024.479955