Vanessa do Nascimento Ferreira 1,2; Luis Otávio Cocito De Araujo 2; Eduardo Linhares Qualharini 3; Elaine G. Vazquez 2; Issa Chaer 4; Mohammad K. Najjar 3*
1, Department of Civil Engineering, University of Lille, France
2, Department of Civil Construction, Polytechnic School, Federal University of Rio de Janeiro, Brazil
3, Environmental Engineering Program, Federal University of Rio de Janeiro, Brazil
4, School of Built Environment and Architecture, London South Bank University, London, UK
E-mail:
mnajjar@poli.ufrj.br
Received: 03/11/2024
Acceptance: 19/12/2024
Available Online: 20/12/2024
Published: 01/01/2025
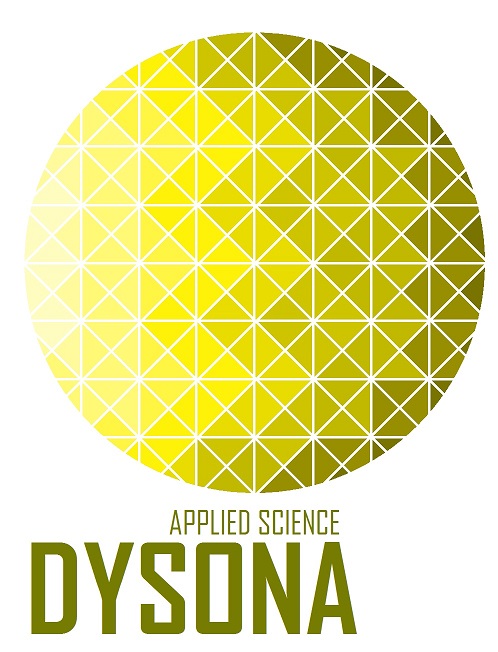
Manuscript link
http://dx.doi.org/10.30493/DAS.2024.486918
Abstract
The growing demand for sustainability and energy efficiency in the construction sector has driven retrofitting as an essential strategy to reduce energy consumption in existing buildings. Despite the availability of numerous efficient building projects, studies specifically focused on energy retrofitting and rehabilitation actions remain scarce. This work evaluates the implementation of building retrofit proposals to increase energy efficiency. Hence, a novel methodological framework is proposed to assess the building retrofit to empower the decision-making process and sustainability of existing buildings. A case study building of the Jean Carasso residence in Bezons, France is applied to evaluate the validity of the proposed framework of this study. The proposed methodological framework of this study consists of four main steps that are characterization and parameters; performance constraints; proposals and alternatives; and quantification of energy benefits. Energy Plus software was used to simulate the energy modelling of existing buildings and evaluate the building retrofit. The case study building consists of two main buildings: B1 and B2. The results indicated that the proposed methods could enhance energy efficiency in building B1 by 65.1% and in building B2 by 68.8%. This work confirms that insulation of external walls is more effective than insulation of internal walls and the simple replacement of the old windows with more efficient windows brings a significant contribution in terms of thermal transmission.
Keywords: Building retrofit, Energy efficiency, Sustainability, Energy consumption
Introduction
The transformation of energy consumption in the construction sector is of utmost importance in the world scene [1][2], particularly in light of the intensified effects of climate change [3][4]. The energy consumption of buildings in developed countries represents 20% to 40% of the total energy use, which is higher than the values related to the industry and transportation sectors in Europe and the United States of America. Since the 1980s, sustainability has gained prominence in civil construction, motivated by the scarcity of resources and inadequate disposal practices. This resulted in the creation of standards for reuse and greater transparency in interventions. At the onset of the 21st century, it became clear that rehabilitating buildings, considering their life cycle, is more advantageous. These buildings, designed according to their time and possessing historical value, began to be viewed differently. The new paradigm prioritizes future maintenance and rehabilitation, regarding both old and new structures as reusable assets [5].
In real estate, rehabilitation consists of renovating a property or a residence without demolishing the structure or architectural style of origin [6]. In this situation, retrofit serves as a viable intervention in rehabilitation. Retrofit is an approach inserted in the set of sustainable solutions due to its focus on reusing existing buildings. These strategies aim to adapt buildings for new uses, conserve urban historical heritage, and improve the performance of buildings in terms of comfort and energy efficiency [7]. A retrofitted building should provide greater comfort and quality of life for its occupants. This goal can achieved by incorporating modern concepts that effectively serve all users [8].
In the construction sector, the designing phase is one of the essential components of the production process [9–11]. During this phase, the design of the building takes place, as well as the specification of materials and construction techniques [12]. Construction project plays a crucial role in promoting the rationalization of construction, ensuring the final quality of the product and its sustainability [13]. Vale [14] classifies retrofit works into four degrees of interventions:
- Rapid Retrofit: facility improvement services and interior cladding;
- Medium Retrofit: includes fast retrofit services, as well as interventions in facades and changes in building installation systems;
- Deep Retrofit: encompasses the previous interventions, in addition to the activities in which there are layout changes (from compartmentalization to the roof structure itself);
- Exceptional Retrofit: occurs mainly in historic buildings or located in protected areas
China, the United States, and Italy are the three countries that developed the most research in the field of building renovation [15]. Several retrofit strategies incorporated into building design are studied globally, evidencing the relevance of rehabilitation in existing buildings to promote energy saving and architectural value. Even if the building design may undergo few significant changes during a retrofit, its crucial role in improving architectural quality and especially in energy savings through energy retrofit should not be underestimated [16][17]. Among the various components of retrofit strategies, the building envelope stands out for its significant contribution to the performance of a building, being fundamental for the quality of natural lighting, thermal comfort, and energy consumption [18]. The building envelope is the set of components that form the physical barrier between the interior and exterior of the building. It includes components such as walls, roofs, windows, doors, and floors [19]. The quality of a building envelope has a major impact on the energy performance of the building [16]. Therefore, a well-designed and constructed envelope is essential to minimize heat losses in winter and heat gain in summer, contributing to a more comfortable indoor environment and the reduction of heating and cooling costs. Ardente et al [20] studied three retrofit cases in which strategies designed as external insulation, and high-efficiency windows resulted in a reduction of up to 50% of energy consumption in heating.
In the context of energy consumption, according to data from the International Energy Agency (IEA), the real estate sector was responsible for (30%) of the final energy demand worldwide. In addition, they contribute to 26% of global emissions, 8% of which are emissions related to the use of gas for heating and cooking, and 18% of electricity consumption [21]. These figures underline the importance of implementing energy efficiency and emission reduction measures in buildings to improve global energy sustainability. Energy retrofits have therefore been implemented to reduce the contribution of existing buildings to greenhouse gas (GHG) emissions and to improve energy performance, ultimately aiming to mitigate climate change [22][23]. Energy retrofit can be classified into three types: “passive”, focusing on reducing building load; “active”, focusing on building system efficiency; and; “renewable”, focusing on on-site energy supply and renewable energy sources [24].
European case studies on energy retrofit of public buildings showed that the most significant results are mainly related to the improvement in envelope thermal insulation (high-efficiency windows and thermal insulation boards) [20]. The replacement of insulation, lighting, and glazing components also offered particularly efficient solutions. Traditional methods of energy renovation are classified as internal thermal insulation and external thermal insulation [6]. Concerning internal insulation of internal walls, it is believed that despite being the most applied practice in the market of retrofit for energy efficiency, this technique does not offer advantageous performances. Exterior wall insulation, on the other hand, offers more favorable results and is more recommended. The authors present the possible interventions as: insulation of external walls; floor slab insulation; roof insulation; replacement of windows; and implementation of heating, hot water, and ventilation systems. Another study found that energy retrofit can reduce energy consumption by (51.2%). However, this efficiency is significantly lower in projects that do not incorporate renewable energy sources, suggesting that there is a limit to reducing energy consumption only with passive measures [25]. Consequently, most energy retrofit projects focus on improvements to the building envelope, which prove to be economically feasible, especially in cold climate regions.
To further strengthen the energy efficiency of building retrofit, the impact of climate change on residential building retrofit in Turkey was examined in two cities with different climate characteristics, conducting dynamic simulations and creating future weather scenarios. This work concluded that the primary energy consumption for heating purposes could be decreased by (36–41%), while primary energy consumption for cooling purposes tripled in the examined cities [26]. Retrofitting methodologies in buildings have emerged as a critical strategy to improve the energy efficiency and sustainability of existing buildings, however, numerous studies have explored retrofitting techniques, where a comprehensive comparative analysis of alternative methodologies and their integration with renewable energy sources remains essential. In these terms, alternative retrofitting methodologies have been designed based on energy efficiency retrofitting focusing on improving the energy performance of buildings through measures such as insulation [27–29], efficient heating, ventilation, and air conditioning (HVAC) systems [30–32], and advanced lighting technologies [33][34]. Other retrofitting methodologies have been designed based on passive design retrofitting by incorporating passive design principles where buildings could harness natural resources such as sunlight and ventilation to reduce energy consumption [35][36]. In literature, several methods and tools have been integrated into the construction industry over the maintenance period to increase energy efficiency such as Building Information Modeling (BIM) (i.e. Autodesk Revit [37], Autodesk Green Building Studio [9][18], and EnergyPlus software [38]), Building Energy Modeling (BEM) [39], Multi-Criteria Decision-Making (MCDM) [40], Life Cycle Assessment (LCA) [41], Internet of Things (IoT) [42], and Robotics in Construction (RC) [43]. Furthermore, several industry standards and certifications have been used at this level of the analysis such as Leadership in Energy and Environmental Design (LEED), Building Research Establishment Environmental Assessment Method (BREEAM), and Energy Star [44].
The concepts of final energy and useful energy assume extreme relevance for the understanding of energy consumption and the implementation of energy efficiency measures [45]. There are several possibilities for energy savings at each stage of the project, depending on the current condition of the building. Accordingly, it is possible to improve the energy performance of a building in several ways, including improvements to the envelope and services such as heating, cooling, ventilation, lighting, domestic hot water, internal equipment, and, in some cases, appliances [46]. The increase in energy consumption and CO2 emissions in the built environment has made energy efficiency strategies a priority in energy policies, leading to the development of new building regulations and certification programs that now necessitate minimum requirements [47]. An experimental analysis of thermal retrofit measures in France was conducted, attributing financing mechanisms, transferability of loans, heating cost savings, and investment costs [48]. The authors found that retrofit measurement such as higher heating cost savings has less interest for landlords and economical thermal insulation for building retrofit should be suggested.
Regarding energy efficiency in buildings, several French and European regulations base efficiency indicators on thermal energy transmission coefficients. The amount of heat that is transmitted through a material or structure per unit time and per unit area, for a unit temperature difference between the two sides of the material is measured by ISO 7345:2018 [49]. In France, the current regulations for energy renovation of buildings incorporate Primary Energy Consumption (PEC) as one of the energy efficiency indicators of the building rehabilitation project in the context of energy renovation, covering the heating, cooling, lighting, domestic hot water production and auxiliary elements (pumps and fans) [50]. For this reason, France applies several regulations and labels such as the Thermal Regulation of Global Existing Building [51] and the BBC Effinergie Renovation 2009 label [52]. This study aims to evaluate the implementation of building retrofit proposals to increase energy efficiency. EnergyPlus software was used as a BIM tool to assess the energy consumption of building retrofit. The proposed framework of this study aims to evaluate the equipment and construction components used before proposing the retrofit measurements for enhancing the thermal insulation in current buildings and avoiding higher heating and cooling costs. the constructive techniques of passive and, eventually, active energy retrofit proposed for the Jean Carasso Residence project will be addressed. Hence, a novel methodological framework to evaluate the retrofitting process in buildings is proposed to increase energy efficiency and empower the decision-making process and sustainability in existing buildings.
Materials and Methods
This study aims to evaluate the implementation of building retrofit proposals to increase energy efficiency. The novelty herein is to propose a methodological framework for evaluating the retrofitted buildings and empowering the decision-making process and sustainability in existing buildings. Hence, a novel framework is proposed (Fig. 1).
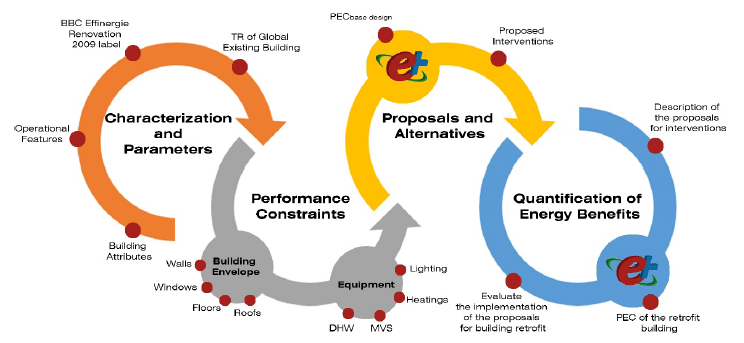
Characterization and parameters
This step is fundamental to the proposed framework for building retrofitting. It involves defining and describing the building attributes, operational features, and building parameters. This step aids in understanding the potential to improve the energy efficiency in the construction project and empower the decision-making process in terms of the selection and implementation of appropriate strategies for building retrofit. Describing the building attributes and operational features includes collecting information related to the physical characteristics such as building orientation and dimensions, functional requirements (i.e. occupancy patterns and capacity), and historical information (i.e. maintenance records and previous retrofits).
Defining building parameters encompasses a deep analysis of the objectives and constraints of the construction project to ensure that the building retrofit is to be conducted following the sustainability goals and operational requirements. At this level of the analysis, it is highly important to define the energy efficiency scope and the applied regulations [53]. This could include the goals of reducing energy consumption, increasing thermal comfort, and complying with environmental regulations. In addition, this stage sets out the sustainable objectives (i.e. indoor environmental quality enhancement, resilience, and social equity), and the technical parameters, indicators, and variables that are to be taken in the analysis. This step aids in developing a clear roadmap for building retrofit to collect the desired outcomes within the identified limitations. In the case study building examined herein, it is fundamental to understand the applied French thermal regulations for the development of the practical analysis of energy retrofit; “Global Existing Building” [51]; and “BBC Effinergie Renovation2009” label [52].
Thermal regulation of global existing building
According to this Thermal Regulation (TR), it is mandatory for existing buildings in France to conduct significant regular renovation. This TR pertains to the renovation of residential and commercial structures under various settings: Floor Area (FA) of the building is more than (1000 m2); the construction date of the building is later than 1st of January, 1948; and The cost of thermal renovation works decided by the owner is more than 25% of the value of the building without the land plot [51]. However, the requirements of the applied TR are defined based on energy analysis of the building, as follows:
- Initial assessment of the building: the initial energy consumption of the building is being calculated to evaluate the initial performance of the building, guide the renovation choices, and estimate the energy savings obtained from the works compared to the previous or worst situations.
- Energy savings: the Primary Energy Consumption (PEC) of the renovated building for heating, ventilation, Domestic Hot Water (DHW), auxiliaries as well as lighting, must be lower than the reference consumption of the same building before retrofit, calculated based on the following equation (Eq. 1):

Where,
: annual Primary Energy Consumption per square meter (kWhPE/m2/year)
H: annual energy consumption for heating per square meter (kWh/m2/year)
V: annual energy consumption for ventilation per square meter (kWh/m2/year)
DHW: annual energy consumption for domestic hot water per square meter (kWh/m2/year)
L: annual energy consumption for lighting per square meter (kWh/m2/year)
A: annual energy consumption for auxiliaries and other equipment per square meter (kWh/m2/year)
- Summer comfort: to lessen the occupant discomfort and the use of air conditioning, the renovated building should ensure acceptable indoor summer comfort.
- Safety measurements: maintaining a minimum performance standard is required for the components (insulation, ventilation, heating system, etc.).
The TR measures thermal efficiency for components that are assembling the building envelope (walls, roofs, floors, windows, and doors) based on the coefficient of thermal transmission (U-value) [54][55]. It is understood that low U-values are desirable to minimize heat loss and improve the energy efficiency of buildings [56].
BBC effinergie renovation 2009 label
To obtain the BBC Effinergie Renovation label, the main requirement is that the energy consumption of the building does not exceed the (PECBBC) of (80 kWhPE/m2/year), multiplied by a coefficient of climatic rigor, as presented in Eq. 2. This coefficient accounts for climatic diversity, incorporating the sum of two additional coefficients: coefficient (a), which pertains to the building’s climatic zone [57], and coefficient (b), which relates to the altitude of the construction site. The coefficient b value is equal to 0.1 if the altitude of the location is between 400 and 800m, and is equal to 0.2 if the altitude of the location is higher than 800 m, while it is equal to 0 if the altitude of the location is lower than 400m [57].

Performance constraints
The energy analysis aims to identify aspects of energy efficiency, assess the current energy consumption of the building, and identify areas of inefficiency, thermal bridges, identifying the areas where thermal bridges occur, which are responsible for significant heat losses in winter and heat gains in summer; insulation, checking the quality of thermal insulation around windows and determining the need for improvements to increase energy efficiency; and regulatory compliance, assessing how well the building meets current thermal regulations and identifying the necessary interventions to ensure compliance. The second phase of the proposed framework involves a thorough assessment of the initial state and performance constraints of the building. This step is crucial to identify potential barriers to retrofit implementation and to develop effective strategies to overcome these challenges. This issue necessitates evaluating the thermal performance and U-value of the construction components of the building envelope such as internal and external walls, floors, roofs, and openings. This relates to the significant role these components play in delineating and recommending the necessary interventions for the energy retrofitting of buildings.
External walls and roofs are responsible for the majority of external thermal loads of buildings due to the heat transfer from the sun, earth, and the built environment [9][58]. Additionally, windows are also a crucial construction components of a building envelope that directly influence energy efficiency, thermal comfort, and natural lighting [59]. The proposed framework of this study highlights the importance of several factors that should be considered when assessing the thermal and energy performance such as the thermal transmission of the window, the solar factor, and the light transmission [60]. The coefficient of thermal transmission (U-value) of Windows indicates the amount of heat that is transmitted through the window [61]. The solar factor refers to the proportion of solar radiation that enters through the window and contributes to internal heating. This factor is important for assessing how windows influence solar heat gain [19]. A high solar factor can increase the internal thermal load during the warm months, while a low factor can limit the desired solar gain in winter [62].
In addition to the components of the building envelope, the energy analysis also includes the assessment of energy-consuming equipment used for heating, lighting, and ventilation purposes [63][64]. These devices influence the overall efficiency of the building, affecting both energy consumption and occupant comfort [65]. Regarding heating, boilers are essential components in buildings, responsible for the heating of rooms, and the production of domestic hot water (DHW) [66]. The analysis of these boilers involves assessing the current efficiency, checking fuel consumption and the ability to effectively provide heating, the state of health of the boilers and the regularity of preventive maintenance, ensuring that they operate in proper conditions, and identifying the technology used, determining whether they are condensing or conventional boilers.
Identification of the proposed interventions
The proposed framework for this study is to suggest measures and alternatives for the building components, including the construction envelope and equipment, after conducting the preliminary evaluation to identify the components that need to be renovated, replaced, or changed. In terms of the building envelope, the proposals may include changing or improving thermal insulation and the U-value of the external and internal walls, slab floors, roofs, and windows. This requires prioritizing the external insulation to improve the thermal performance and minimize thermal bridging [67], replacing existing windows with high-performance and energy-efficient windows [68], and evaluating and monitoring the infiltration rate in the building envelope, taking into consideration gaps, cracks, and penetrations [69].
At the equipment level, this step requires conducting the required maintenance and replacing the Mechanical Ventilation System (MVS), storage tanks for the DHW, boilers, lighting system, and all other heating and cooling systems. This means replacing outdated equipment with high-efficiency systems [70], optimizing the operation systems and reducing energy consumption by implementing advanced control systems [71], and upgrading ventilation systems to reduce energy losses and improve indoor air quality [72]. Besides, it is highly important to evaluate the lighting systems such as optimizing the lighting levels, installing lighting controls, and reducing unnecessary energy use [73]. This step necessitates careful consideration of the building materials, construction components, and energy equipment available in the local construction market of the construction project to implement a well-planned retrofit strategy that could help achieve significant energy savings, improve energy efficiency in buildings, and empower the decision-making process and sustainability in the built environment.
Quantification of energy benefits
The development of the proposed framework of this study has been established based on several criteria and references related to real-world applications, focusing on practical applications of energy efficiency strategies and providing actionable insights for building designers and operators. Besides, it takes into consideration the state-of-the-art tools, using EnergyPlus, as a well-validated and widely used building energy simulation tool to accurately model building energy performance [74]. This software considers worldwide climate classification and building characteristics [75].
This is the most critical step in the proposed framework, where the energy modeling should be simulated in EnergyPlus software as a BIM tool. Creating an input file in EnergyPlus requires the available methods to create files such as Input Data Files Editor, BLAST Translator, DOE-2 Translator, and Hand Editing [76-79]. This study will consider the Input Data Files Editor methods taking into consideration the input data such as building geometry, construction materials, energy systems, occupancy and schedules, and weather data. Such data is required to build up the primary file that defines all the building parameters [76][78].
Based on the design proposals, it is essential to analyze the energy gains of the suggested strategies, both in isolation and as a whole. The individual evaluation of each strategy allows identifying the specific impacts of each intervention, enabling prioritization. However, the overall analysis is crucial to understand the synergy between the different proposals and the effectiveness of the combined actions in the context of the energy retrofit. The dynamic energy simulation method is performed through EnergyPlus, associated with data that describes the geometry of buildings and meteorological data allow the calculation engine to estimate energy consumption for the existing buildings before and after retrofitting.
The last step of the proposed framework for this study requires carrying out a verification analysis to ensure that the interventions implemented meet the objectives and requirements defined at the beginning of the project. This verification involves measuring the results of simulations and comparing them with the set goals. In other words, this step means to compare the PEC of the evaluated building before and after the retrofitting works and compare with the PECBBC and PECTR. Adjustments and corrections may be done as necessary to ensure compliance with energy performance criteria. This phase is decisive for the approval or not of the proposed measures.
Case study
This work assesses and evaluates a real case study building in France to validate the resolution of the proposed framework. Hence, the practical application is applied as follows:
Characterization and parameters
The case study building assessed in the present work is a social residence known as “Jean Carasso” located in the city of Bezons in France. It consists of two buildings with a total of 244 residential units (Fig. 2). The main building, identified as B1, was built in the early 1960’s. The first renovation, occurred in 1994, included the modernization of rooms and common areas, where the building was expanded from three floors to five floors. Its capacity was 157 single rooms of 12 to 16 m2, without a private bathroom or individual kitchen. However, the architectural project dedicated a small common area for each group of three to eight rooms, provided with a bathroom and a kitchen. Furthermore, in 1994, the social residence of Jean Carasso was completed with the construction of a new curved building, identified as a B2. B2 is constituted of three floors and an underground car parking, with a capacity of 82 single rooms of 12 to 16 m2.
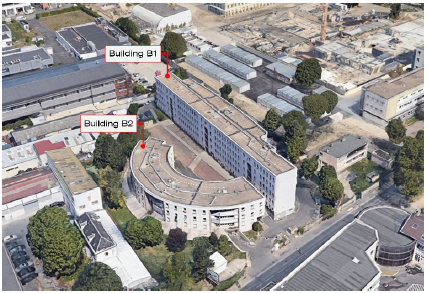
The social residence of Jean Carasso has always operated at the maximum capacity of users. However, due to new energy requirements, it faced a lack of standards and comfort for its residents. To maintain and improve the attractiveness of the enterprise, the project was developed with a focus on energy rehabilitation. The objective of the rehabilitation is to transform the existing 244 residential units into 211 individual studios (T1, T1′, and T1_bis), distributed between the two buildings (B1 and B2). The total surface area of B1 and B2 is 2825.67 m2 and 1414.88 m2, respectively. The typology T1 has an area of 18 m2, T1′ has an area of 24 m2, and T1_bis has an area of 30 m2. The quantity of each typology in buildings B1 and B2 is presented in Table 1. The Thermal Surface Area (TSA) was calculated from the total outside-to-outside built-up of external facades. In these terms, it can be noted that B1 and B2 has a TSA of 3917.85 m2 and 1645.29 m2, respectively.
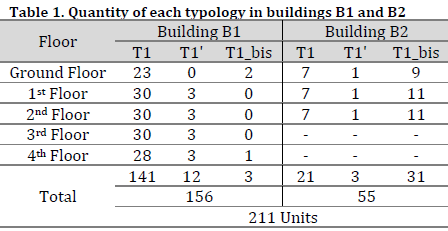
The main objectives for the energy retrofit of the social residence of Jean Carasso are based on compliance with the French TR “Global Existing Building” and the “BBC Effinergie Renovation 2009” label. The knowledge of these regulations facilitates the identification of best practices and applicable technologies, ensuring that retrofit for energy efficiency meets the requirements of energy efficiency and promoting a rehabilitation that respects both the built heritage and the environmental and economic goals of the country.
Initial state and general features
The annual PEC of the case study has been evaluated through several technical visits and conducting various analyses of buildings B1 and B2. It was noted that the case study building consumed 1543581.54 kWhPE/year. This value was used for heating (729084.86 kWhPE/year), ventilation (176646.61 kWhPE/year), DHW (232110.43 kWhPE/year), lighting (396506.21 kWhPE/year), and auxiliary equipments (9233.43 kWhPE/year). These values justify the necessity to address the heating and DWH systems, which account for around (62.27%) of the total annual PEC of the building. The ventilation and lighting systems account for 11.44% and 25.69%, respectively. At this level of the analysis, it is important to understand the characteristics of the building envelope and the features of the equipment used in the case study as a prior step to suggest interventions and potential improvements, such as slabs, roofs, and internal and external walls (Table 2).
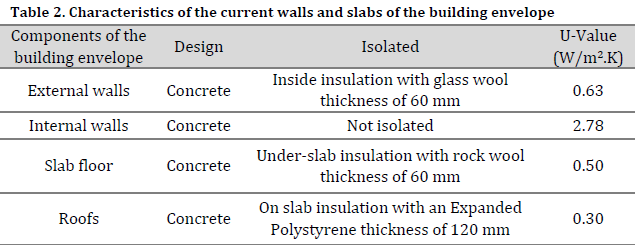
It can be noted that internal walls collaborate significantly for heat exchange between environments, while the roofs with expanded polystyrene insulation have low heat transmission, indicating high thermal insulation. Furthermore, it is observed that the glass wool used in external walls is likely to be a less thermally resistant insulator when compared to the other options available in the local market of construction components in France (Table 2). The thermal transmission of slab floors must also be considered. This can be attributed to its high U-value reflection compared to other components. Thus, the application of continuous insulation could improve the transmission performance of the building envelope. In terms of analyzing the thermal performance of the windows used in buildings B1 and B2, the existing windows were structured with double glazing and shutters in PVC, with a U-value equal to 2.9 W/m2.K.
The boilers used for heating and DHW production use a condensing production system. There are mainly two gas boilers located in the boiler house in the basement of building B1, with a rated power of 403 kW/boiler (Table 3).
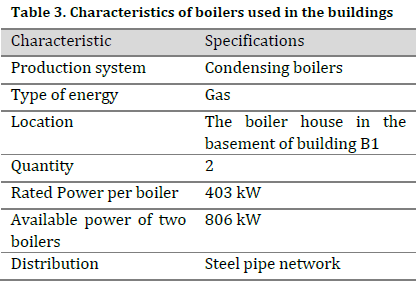
Other energy consumption systems should also be evaluated such as ventilation and lighting systems, since being planned to be completely replaced. It can be observed that no changes or maintenance have been done for these systems for a long-standing period. These systems combined are responsible for 37.13% of the total annual PEC of buildings B1 and B2 before the retrofit. Meeting the needs of 241 residential units for DHW and heating purposes requires evaluating the capacity and rated power of the boilers. Observing the energy consumption in the case study building, it can be noted that the total power required for heating and DHW is 722 kW. Comparing these values with the currently available power of the two boilers (806 kW), it can be understood that there is no need to change the current boilers used in the building. However, the existing ventilation and lighting systems should be evaluated and replaced by developed and recent appliances.
Results and Discussion
The PEC values of the base design of the case study building were simulated and evaluated in Energy Plus (versions 6.0.0.023), highlighting that building B1 could consume up to 271.99 kWhPE/m2/year whereas the PEC value of building B2 is around 299.97 kWhPE/m2/year.
The next step was to estimate the reference PEC values of the examined case study based on the TR of Global Existing Building [51] and the label of BBC Effinergie Renovation 2009 [52]. In terms of the TR of Global Existing Building and applying Eq. 1, it can be realized that the reference PECTR values of buildings B1 and B2 are 137 kWhPE/m2/year and 130 kWhPE/m2/year, respectively.
In terms of the label of BBC Effinergie Renovation 2009 and applying Eq. 2, it can be noted that the value of coefficient (a) is equal to 1.3 for the region of the city of Bezons (Region H1a) [57], while the value of coefficient (b) is equal to (0), since the altitude of the region is less than 400 meters. Hence, the reference PECBBC value should be 104 kWhPE/m2/year for each building.
This means that the existing case study buildings B1 and B2 are exceeding the referenced values based on the PECTR and PECBBC. Hence, a list of proposals for interventions is listed to enhance the energy efficiency in the building.
Proposals for interventions
Clarifying the proposals for interventions was based on the construction components of the building envelope and the equipment used in the buildings. In these terms, the relative surface area of the external construction components that are assembling the building envelope (i.e. external walls, windows, roofs, and floors) of the case study, which could aid in estimating the representativeness of the components in the thermal losses of the envelope (Fig. 3). Walls represent up to 42% of the total external components of the building envelope, while the floors represent 9% only. In fact, integrating phase change materials into building envelopes, especially external walls roofs and, can effectively enhance thermal comfort and energy consumption [80].
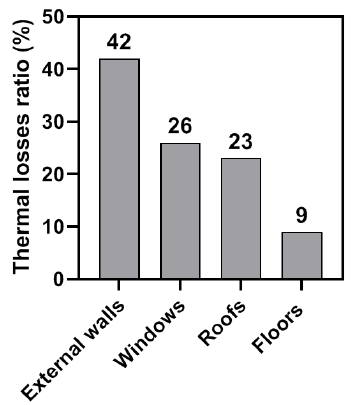
Consequently, few solutions were proposed for the envelope. These changes included changing the insulation of the external walls, slab floor, and windows, as well as for the equipment such as the storage tank of DHW, MVS, and artificial lighting, as follows:
- External walls: use an outside insulation-type mineral wool thickness of 160 mm (U-value equal to 0.19 W/m2.K).
- Internal walls: use the base design model without changes.
- Slab floor: use under-slab insulation with a rock wool thickness of 120 mm (U-value equal to 0.29 W/m2.K).
- Roofs: use the base design model without changes, as it appeared in a good state.
- Windows: use windows structured with double glazing and shutters in PVC (U-value equal to 1.4 W/m2.K), and light transmission equal to 0.54.
- Boilers: use the base design model without changes.
- Storage tank for DHW: use a storage tank with a capacity of 1500 liters, tank power of 145 kW, and heat exchanger power of 387 kW.
- Equipment of MVS: use hygro-adjustable single flow MVS with a flow rate of 12000 m3/h and a power of 369 W in building B1, and a flow rate of 5000 m3/h and a power of 118 W in building B2.
- Artificial lighting: use LED lamps with a timer or presence detector in common areas such as corridors, technical areas, garages, and other environments outside the residential units, prioritizing direct lighting and avoiding indirect lighting.
Quantifying energy gains
With the design assumptions presented in the proposal session and simulated in Energy Plus, it was possible to establish the weighted design thermal transmission coefficient for all elements of the rehabilitated buildings envelope, representing a PEC value of 94.87 and 93.74 kWhPE/m2/year for B1 B2, respectively (Fig. 4). By comparing these values with the PEC values of the base design of the buildings, it can be noted that the energy efficiency could be enhanced by 65.1% in building B1, and 68.8% in building B2. When evaluating the PEC values of the project after the energy retrofit proposals, it can be observed that the proposals are validated concerning the established parameters. For compliance with the TR of Global Existing Building (137 and 130 kWhPE/m2/year for buildings B1 and B2, respectively) and the label of BBC Effinergie Renovation 2009 (104 kWhPE/m2/year for both buildings), since both buildings meet the maximum reference consumption limits.
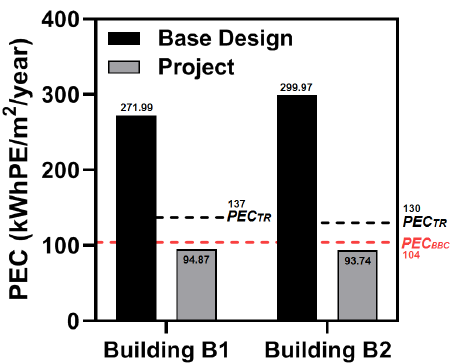
This endeavor resulted in a reduction of the building’s permeability, specifically regarding air penetration. Additionally, it achieved improvements in fundamental energy use, which underpins all equipment decisions, hence enhancing building efficiency. Other suggestions might be applied to increase energy efficiency of the studied buildings including incorporating vegetated surfaces on roofs [81] and upgrading ventilation systems (Table 4).
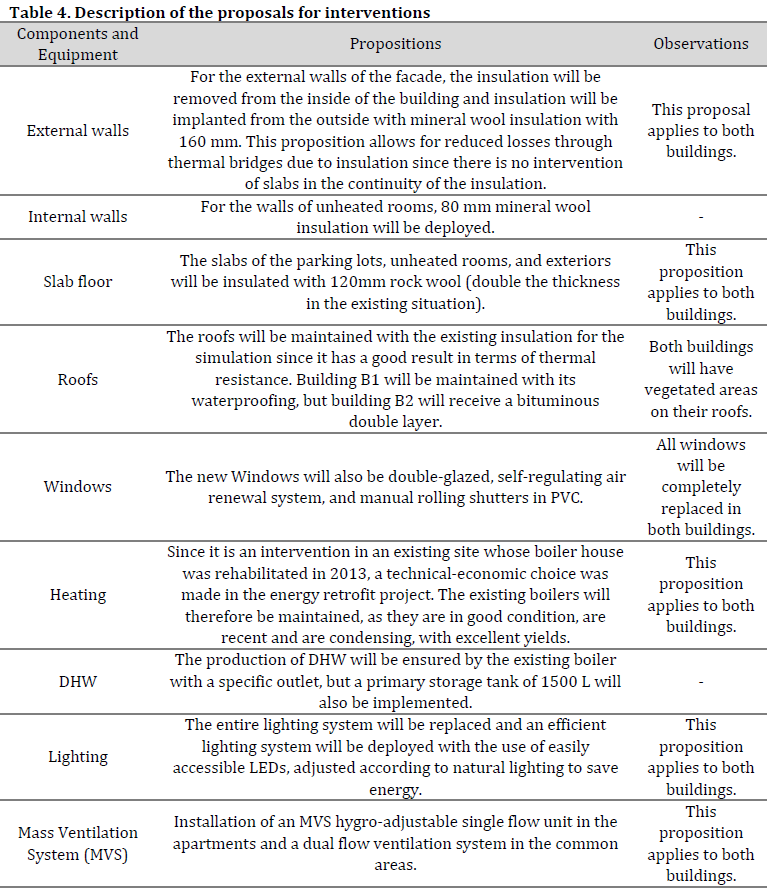
Evaluating the obtained energy savings for the case study building after implementing retrofitting measures, taking into consideration the key interventions for external wall insulation, improved window systems, and adjustments to building services, it can be observed that the findings align with French TR and the BBC Effinergie Renovation 2009 label, underscoring the compliance and practicality of the proposed retrofitting approach and enabling informed decision-making for building designers and policymakers. In such circumstances, the proposed retrofitting framework for this study could achieve remarkable cost-effectiveness based on long-time energy savings and payback period. Moreover, applying the proposed framework on building retrofit could improve the indoor air quality and the sustainable materials used for external and internal insulation towards reducing the environmental impacts over the entire lifespan of buildings and protecting the built environment.
Conclusions
This study introduces an innovative methodological approach for evaluating building retrofits to enhance energy efficiency. The methodology has four primary steps: characterization and parameters, performance limits, proposals and alternatives, and quantification of energy gains. A case study of the Jean Carasso house in France is employed to assess the framework’s validity. The research indicated that passive energy retrofits markedly decrease energy consumption. Moreover, the insulation of external walls proved to be more efficient than that of internal walls, and basic window replacements substantially affect thermal transmission. The application of the framework to the case study buildings yielded substantial energy efficiency enhancements, with buildings B1 and B2 achieving increases of 65.1% and 68.8%, respectively.
Conducting similar studies in diverse climatic regions in France and worldwide based on expanding the geographical scope could be a recommendation for future studies to assess the effectiveness of retrofit strategies of the proposed framework across varying contexts and identify transferable solutions. Furthermore, this study indicates employing sophisticated simulation tools as another recommendation for future studies to optimize retrofit strategies and assess long-term energy performance using innovative technologies such as smart grids, renewable energy systems, and advanced building materials to increase energy efficiency and sustainability in building retrofit. Besides, integrating retrofit strategies with other sustainable methods such as Life Cycle Assessment and Multi-Criteria Decision-Making could be another recommendation for future analysis. Addressing such recommendations could contribute to advancing energy-efficient building retrofits and endorsing sustainable urban development in the built environment.
Exploring the integration of renewable energy systems such as solar panels with passive retrofitting methodologies could be considered to significantly enhance the holistic nature of the proposed framework and provide valuable insights for sustainable building retrofitting practices. Such a recommendation could encourage the adoption of renewable energy systems, particularly in social housing contexts, taking into consideration the financial incentives, regulatory frameworks, and public-private partnerships. Furthermore, integrating renewable energy systems and passive measures could enhance accessibility and incorporate clear visualizations to illustrate the energy and cost benefits.
Acknowledgments
The authors would like to acknowledge the support of Fundação Carlos Chagas Filho de Amparo à Pesquisa do Estado do Rio de Janeiro (FAPERJ E-26/210.950/2024 (295973)).
References
- Najjar MK, De Araujo LO, Oladimeji O, Khalas M, Figueiredo KV, Boer D, Soares CA, Haddad A. Influence of Ventilation Openings on the Energy Efficiency of Metal Frame Modular Constructions in Brazil Using BIM. Eng. 2023;4(2):1635–54. DOI
- Berardi U. Sustainability assessment in the construction sector: rating systems and rated buildings. Sustain. Dev. 2012;20(6):411-24. DOI
- Hafez FS, Sa’di B, Safa-Gamal M, Taufiq-Yap YH, Alrifaey M, Seyedmahmoudian M, Stojcevski A, Horan B, Mekhilef S. Energy efficiency in sustainable buildings: a systematic review with taxonomy, challenges, motivations, methodological aspects, recommendations, and pathways for future research. Energy Strategy Rev. 2023;45:101013. DOI
- Billio M, Casarin R, Costola M, Veggente V. Learning from experts: Energy efficiency in residential buildings. Energy Econ. 2024;136:107650. DOI
- Qualharini EL. Evolução da reabilitação predial no século XXI. Gestão e Gerenciamento. 2019;6(6).
- Beddiar K, Chazal P, Ziour R. Massification de la rénovation énergétique: accélérer et optimiser la rénovation énergétique grâce aux outils numériques et industriels. Dunod. 2022.
- Pietrangeli I, Mazzuto G, Ciarapica FE, Bevilacqua M. Smart retrofit: an innovative and sustainable solution. Machines. 2023;11(5):523. DOI
- Ortiz M, Itard L, Bluyssen PM. Indoor environmental quality related risk factors with energy-efficient retrofitting of housing: A literature review. Energy Build. 2020;221:110102. DOI
- Najjar M, Figueiredo K, Palumbo M, Haddad A. Integration of BIM and LCA: Evaluating the environmental impacts of building materials at an early stage of designing a typical office building. J. Build. Eng. 2017;14:115-26. DOI
- Najjar MK. Optimizing the Sustainable Decision-Making Process Towards Improving Energy Performance Over the Entire Life Cycle of Buildings. PhD thesis Federal University of Rio de Janeiro. 2019.
- Quintanilha BP, Almasri A, Amario M, Qualharini EL, Najjar M. Avaliação do desempenho térmico de fachadas verdes para edifícios energeticamente eficientes usando bim. Simpósio nacional de gestão e engenharia urbana. 2023;4(00). DOI
- Gurupatham SV, Jayasinghe C, Perera P, Lepakshi R. Building material selection framework for tropical climatic conditions: Eco-design-based approach. Green Technol. Sustain. 2024;2(3):100103. DOI
- Borges D B, Soares C AP, Najjar M, Costa BBF da, Tam VWY, Haddad AN. Project success and critical success factors of construction projects from the perspective of a multicultural team: a case study in Guyana. Int. J. Constr. Manag. 2024;1–15. DOI
- Vale MS. Diretrizes para racionalização e atualização das edificações: Segundo o conceito da qualidade e sobre a ótica do retrofit. Federal University of Rio de Janeiro: FAU. 2006.
- He C, Hou Y, Ding L, Li P. Visualized literature review on sustainable building renovation. J. Build. Eng. 2021;44:102622. DOI
- Rabani M, Madessa HB, Nord N. A state-of-art review of retrofit interventions in buildings towards nearly zero energy level. Energy Procedia. 2017;134:317–26. DOI
- Neme C, Gottstein M, Hamilton B. Residential efficiency retrofits: a roadmap for the future. Regulatory Assistance Project, Brussels, Belgium. 2011.
- Najjar M, Figueiredo K, Hammad AWA, Haddad A. Integrated optimization with building information modeling and life cycle assessment for generating energy efficient buildings. Appl. Energy. 2019;250:1366–82. DOI
- Najjar MK, Figueiredo K, Hammad AWA, Tam VWY, Evangelista ACJ, Haddad A. A framework to estimate heat energy loss in building operation. J. Clean. Prod. 2019;235:789–800. DOI
- Ardente F, Beccali M, Cellura M, Mistretta M. Energy and environmental benefits in public buildings as a result of retrofit actions. Renew. Sustain. Energy Rev. 2011;15(1):460–70. DOI
- International Energy Agency. Final energy consumption of buildings relative to other sectors. IEA. 2023.
- Pombo O, Allacker K, Rivela B, Neila J. Sustainability assessment of energy saving measures: A multi-criteria approach for residential buildings retrofitting—A case study of the Spanish housing stock. Energy Build. 2016;116:384–94. DOI
- Liyanage DR, Hewage K, Hussain SA, Razi F, Sadiq R. Climate adaptation of existing buildings: A critical review on planning energy retrofit strategies for future climate. Renew. Sustain. Energy Rev. 2024;199:114476. DOI
- Shen P, Braham W, Yi Y. The feasibility and importance of considering climate change impacts in building retrofit analysis. Appl. Energy. 2019;233–234:254–70. DOI
- Bjelland D, Brozovsky J, Hrynyszyn BD. Systematic review: Upscaling energy retrofitting to the multi-building level. Renew. Sustain. Energy Rev. 2024;198:114402. DOI
- Tomrukcu G, Ashrafian T. Climate-resilient building energy efficiency retrofit: Evaluating climate change impacts on residential buildings. Energy Build. 2024;316:114315. DOI
- Kallioras S, Bournas D, Koutas L, Molina FJ. Integrated seismic and energy retrofitting of masonry-infilled RC buildings with textile-reinforced mortar and thermal insulation: Full-scale tests on a five-story prototype. J. Build. Eng. 2024;98:111006. DOI
- Yun WS, Ryu W, Lee D, Seo H. Energy-saving potential estimation of retrofitting aged buildings considering external wall insulation degradation. J. Build. Eng. 2024;94:110022. DOI
- Palumbo M, Simo M, Fernández AI, Bosch M. Case study VI: Insulation materials choice in building retrofitting: the influence of social determinants in the Spanish context. Materials Selection for Sustainability in the Built Environment. 2024;465–84. DOI
- Kabbara Z, Jorens S, Seuntjens O, Verhaert I. Simulation-based optimization method for retrofitting HVAC ductwork design. Energy Build. 2024;307:113991. DOI
- Singh N, Permana I, Agharid AP, Wang FJ. Retrofitting HVAC systems for enhanced energy performance and resilience in university data center room. J. Build. Eng. 2024;95:110162. DOI
- Cui W, Liu G, Wang Y, Li K. Integrated optimization of the building envelope and the HVAC system in office building retrofitting. Case Stud. Therm. Eng. 2024;62:105185. DOI
- Belany P, Hrabovsky P, Kolkova Z. Combination of lighting retrofit and life cycle cost analysis for energy efficiency improvement in buildings. Energy Reports. 2021;7:2470–83. DOI
- Bonomolo M, Baglivo C, Bianco G, Maria Congedo P, Beccali M. Cost optimal analysis of lighting retrofit scenarios in educational buildings in Italy. Energy Procedia. 2017;126:171–8. DOI
- Fine JP, Touchie MF. Evaluating ventilation system retrofits for high-rise residential buildings using a CONTAM model. Build. Environ. 2021;205:108292. DOI
- Carlsson M, Touchie M, Richman R. Investigating the potential impact of a compartmentalization and ventilation system retrofit strategy on energy use in high-rise residential buildings. Energy Build. 2019;199:20–8. DOI
- Najjar MK, Franco de Carvalho Willcox L, Khalas M, Da Costa B, Haddad A, Boer D. BIM and Experimental Design Analysis Toward Sustainable Energy Efficiency in Buildings: A Modular Construction Case Study. Proceedings of CIRMARE 2023. 2023;483–95. DOI
- Ren Y, Ogura H. Feasibility study with EnergyPlus simulation for solar chemical heat pump unit introduced into building as next-generation energy supply system. Energy Build. 2024;313:114256. DOI
- Guízar Dena AJ, García Hípola M del C, Fernández Bandera C. Optimization testing for the modeling and characterization of three-dimensional elements to enhance interoperability from building information modeling (BIM) to building energy modeling (BEM). Energy Build. 2024;317:114394. DOI
- Filho MV, da Costa BB, Najjar M, Figueiredo KV, de Mendonça MB, Haddad AN. Sustainability assessment of a low-income building: A BIM-LCSA-FAHP-based analysis. Buildings. 2022;12(2):181. DOI
- Haddad AN, Sedrez MM, Najjar MK, Hammad AWA, Soares CAP. Characterising Embodied Energy in Construction Activities Using Energy Inventory Life Cycle Assessment Method. Buildings. 2022;13(1):52. DOI
- García-Monge M, Zalba B, Casas R, Cano E, Guillén-Lambea S, López-Mesa B, Martínez I. Is IoT monitoring key to improve building energy efficiency? Case study of a smart campus in Spain. Energy Build. 2023;285:112882. DOI
- Al Masri A, Barzellay F. da Costa B, Vasco D, Boer D, N. Haddad A, K. Najjar M. Roles of Robotics in Architectural and Engineering Construction Industries: Review and Future Trends. J. Build. Des. Environ. 2024;3(1):0–0. DOI
- Figueiredo K, Najjar MK, Hammad AWA, Haddad AN. Standards and legal regulations regarding sustainable construction. Materials Selection for Sustainability in the Built Environment. Woodhead Publishing. 2024;117–30. DOI
- Ramos TF, Naves AX, Boer D, Haddad AN, Najjar MK. Sustainability Analysis of Environmental Comfort and Building Information Modeling in Buildings: State of the Art and Future Trends. Eng. 2024;5(3):1534–65. DOI
- Energy Efficiency in Building Renovation. Handbook of Energy Efficiency in Buildings. 2019;675–810. DOI
- Pérez-Lombard L, Ortiz J, Pout C. A review on buildings energy consumption information. Energy Build. 2008;40(3):394–8. DOI
- Fanghella V, Guetlein MC, Schleich J, Sebi C. Preferences on financing mechanisms for thermal retrofit measures in multi-owner buildings: A discrete choice experiment with landlords and owner-occupiers in France. Resour. Energy Econ. 2023;74:101392. DOI
- International Standard ISO 7345. Thermal performance of buildings and building components — Physical quantities and definitions. ISO. 2018.
- Statista. Primary energy consumption in France from 2000 to 2023. BP. 2024.
- Majou G. Arrêté du 22 mars 2017 modifiant l’arrêté du 3 mai 2007 relatif aux caractéristiques thermiques et à la performance énergétique des bâtiments existants–Développement durable & responsabilité sociétale dans l’enseignement supérieur, les formations et la recherche. 2017.
- Queyranne JP. -Jack, Réuissir un projet Bâtiment Basse Consommation en renovation. Paris. 2011.
- Ma Z, Cooper P, Daly D, Ledo L. Existing building retrofits: Methodology and state-of-the-art. Energy Build. 2012;55:889–902. DOI
- Najjar MK, Rosa AC, Hammad AW, Vazquez E, Evangelista AC, Tam VW, Haddad A. A regression-based framework to examine thermal loads of buildings. J. Clean. Prod. 2021;292:126021 DOI
- Bülow-Hübe H. Energy-efficient window systems-effects on energy use and daylight in buildings. Doctoral Dissertation. Lund University, Lund Institute of Technology. 2001.
- Najjar M, Figueiredo K, Haddad A. Increasing energy efficiency of building envelopes towards nearly zero energy buildings integrating BIM and LCA. LA SDEWES 2018. 2018.
- Sénova. Enfin tout comprendre sur les labels « BBC Rénovation 2009 », « BBC Effinergie Rénovation » et « Effinergie Rénovation » pour les logements! Sénova. 2013.
- González J, Soares C, Najjar M, Haddad A. BIM and BEM Methodologies Integration in Energy-Efficient Buildings Using Experimental Design. Buildings. 2021;11(10):491. DOI
- Panigrahi B, Vishwakarma P, Saputra GBAA. Dynamically transparent microfluidic layers for adaptive thermal management and energy efficiency in smart façades and windows. Energy Build. 2024;324:114884. DOI
- Olu-Ajayi R, Alaka H, Egwim C, Grishikashvili K. Comprehensive Analysis of Influencing Factors on Building Energy Performance and Strategic Insights for Sustainable Development: A Systematic Literature Review. Sustainability. 2024;16(12):5170. DOI
- Cuce E. Accurate and reliable U -value assessment of argon-filled double glazed windows: A numerical and experimental investigation. Energy Build. 2018;171:100–6. DOI
- Zheng N, Wang Q, Ding X, Zhang H, Duan L, Wang X, Zhou Y, Sun M, Desideri U. Techno-economic analysis of a novel solar-based polygeneration system integrated with vanadium redox flow battery and thermal energy storage considering robust source-load response. Appl. Energy. 2024;376:124288. DOI
- Masy G, André P. Total energy use in air conditioned buildings: Analysis of main influencing factors. HVAC&R Research. 2012;18(1-2):21-36. DOI
- De Arruda RN, Figueiredo K, Vasco DA, Haddad A, Najjar MK. Cost-benefit analysis of solar energy integration in buildings: a case study of affordable housing in Brazil. Front. Built Environ. 2023;9. DOI
- Melgaard SP, Johra H, Nyborg VØ, Marszal-Pomianowska A, Jensen RL, Kantas C, Larsen OK, Hu Y, Frandsen KM, Larsen TS, Svidt K. Detailed operational building data for six office rooms in Denmark: Occupancy, indoor environment, heating, ventilation, lighting and room control monitoring with sub-hourly temporal resolution. Data Brief. 2024;54:110326. DOI
- Haddad AN, Silva AB, Hammad AWA, Najjar MK, Vazquez EG, Tam VWY. An integrated approach of building information modelling and life cycle assessment (BIM-LCA) for gas and solar water heating systems. Int. J. Constr. Manag. 2022;23(14):2452–68. DOI
- Vaseghi A, Capano CD. Comparative analysis of insulation strategies for improving thermal performance of wall to parkade suspended slab. Energy Built Environ. 2025;6(1):147–60. DOI
- Chen J, Xiao L, Zhang K, Wu T, Luo X, Wang Y, Peng P. First principles investigation on Ca La1-B6 as the solar radiation shielding material for energy-efficient windows. Mater. Lett. 2024;372:136932. DOI
- Zheng S, Song X, Duanmu L, Xue Y, Wang Y, Yang X. Research on the air-infiltration rate shelter coefficient of building complexes based on building parameter clustering. J. Build. Eng. 2024;91:109615. DOI
- de Souza I, Rosa A, Vidal M, Najjar M, Hammad A, Haddad A. Information Technologies in Complex Socio-Technical Systems Based on Functional Variability: A Case Study on HVAC Maintenance Work Orders. Appl. Sci. 2021;11(3):1049. DOI
- Ruano MV, Ribes J, Ruiz-Martinez A, Seco A, Robles A. An advanced control system for nitrogen removal and energy consumption optimization in full-scale wastewater treatment plants. J. Water Process Eng. 2024;57:104705. DOI
- López Plazas F, Sáenz de Tejada C. Natural ventilation to improve indoor air quality (IAQ) in existing homes: The development of health-based and context-specific user guidelines. Energy Build. 2024;314:114248. DOI
- Zhang X, Wang J, Zhou Y, Wang H, Xie N, Chen D. A multi-objective optimization method for enclosed-space lighting design based on MOPSO. Build. Environ. 2024;250:111185. DOI
- Chuah JW, Raghunathan A, Jha NK. ROBESim: A retrofit-oriented building energy simulator based on EnergyPlus. Energy Build. 2013;66:88–103. DOI
- Martin GL, Monfet D. Comparison of EnergyPlus inside surfaces convective heat transfer coefficients algorithms for energy modelling of high-density controlled environment agriculture. Energy Build. 2024;319:114568. DOI
- Crawley DB, Lawrie LK, Winkelmann FC, Buhl WF, Huang YJ, Pedersen CO, Strand RK, Liesen RJ, Fisher DE, Witte MJ, Glazer J. EnergyPlus: creating a new-generation building energy simulation program. Energy Build. 2001;33(4):319-31. DOI
- Atam E. Current software barriers to advanced model-based control design for energy-efficient buildings. Renew. Sustain. Energy Rev. 2017;73:1031–40. DOI
- Esteves D, Silva J, Martins L, Teixeira J, Teixeira S. Building Energy Performance: Comparison Between EnergyPlus and Other Certified Tools. Computational Science and Its Applications – ICCSA 2021. 2021;493–503. DOI
- Lee GJ, Lee JW, Choi HW, Kim S, Kang YT. CO2 thermochemical sorption battery driven by low temperature heat source for plus energy building application. Appl. Energy. 2025;377:124401. DOI
- Al-Yasiri Q, Szabó M. Incorporation of phase change materials into building envelope for thermal comfort and energy saving: A comprehensive analysis. J. Build. Eng. 2021;36:102122. DOI
- Manso M, Gomes JC, Silva PD, Virtudes AL, Delgado FM. Modular system design for vegetated surfaces: a proposal for energy-efficient buildings. BESS-SB13 CALIFORNIA: Advancing Towards Net Zero. 2013.
Cite this article:
do Nascimento Ferreira, V., Cocito De Araujo, L. O., Linhares Qualharini, E., Vazquez, E. G., Chaer, I., Najjar, M. K. Building retrofit for energy efficiency in existing buildings: A case study of a social residential building in France. DYSONA – Applied Science, 2024;6(1): 223-238. doi: 10.30493/das.2024.486918.1161