Pratiksha Patil 1*; Dr Nisha Shah 1
1, Department of Life Sciences, University of Mumbai, Vidya Nagari, Kalina, Santacruz (E), Mumbai-400098, India
E-mail:
pratikshapatil002.pdp@gmail.com
Received: 15/07/2022
Acceptance: 20/09/2022
Available Online: 22/09/2022
Published: 01/10/2022
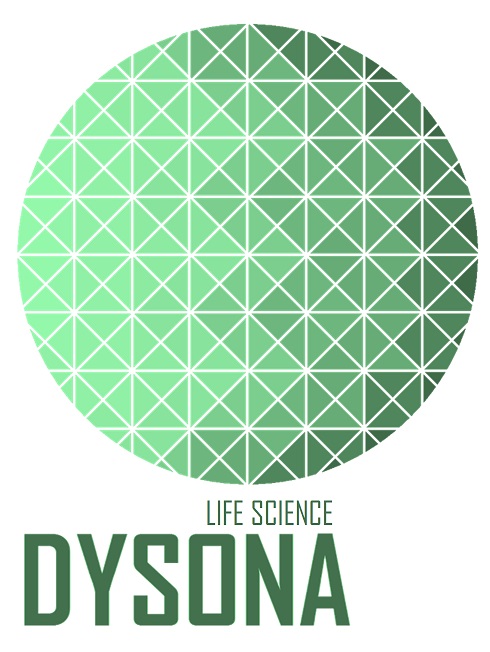
Manuscript link
http://dx.doi.org/10.30493/DLS.2022.351879
Abstract
Prostate carcinoma (PCA) is amongst the most prevalent types of cancer and the underlying cause of death in males across the globe. Despite various restorative advancements, cancer remains an incurable disease. Recent research has emphasized the chemo-preventive effects of phytonutrients and their ability to prevent malignancy. This study aims to identify the potential antagonistic affinity of phytochemicals present in Morinda citrifolia against PCA. Six inhibitory phytochemicals were analyzed against human androgen receptors (PDB: 3RLJ, 5T8J, 5VO4) and prostate-specific antigens (PDB: 2ZCH, 2ZCK, 2ZCL). The ADME/T analysis showed that most phytochemicals had suitable pharmacokinetic properties. Although damnacanthal, morindone, and oleic acid had high gastrointestinal absorption potentials, all tested molecules failed the oral bioavailability test. The ligands’ affinity for a targeted protein was predicted via molecular docking simulation. Docking results showed that among the six small molecules, oleic acid had the strongest binding affinity with prostate-specific antigen (PSA) enzymes with active site chain A (auth P) (2ZCH: ΔG = -7.59 kJ/mol, 2ZCK: ΔG = -8.41 kJ/mol, and 2ZCL: ΔG = -8.27 kJ/mol). On the other hand, alizarin, lauric acid, and morindone resulted in more stable complexes with androgen receptor (AR) active site chain A. This data hints that further studies can be conducted to validate the potent anticancer effects of these molecules against prostatic carcinoma.
Keywords: Morinda citrifolia, In silico, Pharmacokinetics, Prostate cancer, Prostate-specific antigen, Androgen receptor
Introduction
Traditional medicinal systems all over the world have long been reliant on plants and their natural components. Throughout time, plants have provided humankind with new methods of healing. Potent drugs can be synthesized straight from natural products by following their synthesis protocol [1].
The rapid growth of chronic diseases such as cancer has been escalating over the past few years and is the most challenging situation for epidemiology in developed and underdeveloped nations. Although various therapeutic advances have been made, cancer remains a treatable but not curable disease [2]. Prostate therapy involves several pharmacological strategies across different target types, but the search for effective strategies is imperative [3].
Prostatic carcinoma (PCA) is one of the most prevalent types of cancer. Early stage detection can significantly increase survival rates, although treatment in its advanced stages is ineffective. New estimates reveal PCA as the leading cause of death in men in 2022 [4]. According to the world health organization, the statistical data on PCA states that it is among the 4th most ubiquitous types of carcinoma globally [5]. Recently, the National Cancer Institute’s SEER program has estimated 268,490 new cases and 34,500 deaths related to PCA, representing 14% of all the new cancer cases and 5.7% of all cancer deaths [6]. PCA is commonly diagnosed in males above 60, and the number of PCA cases is higher in western countries, Africa, America, and European Caucasians than in Asian countries. The American Cancer Society (ACS) estimates that one in nine men will be diagnosed with PCA over their lives, and one in forty-one men will die from PCA [7]. The underlying factor for PCA is linked to the type of food consumed. Fat stimulates the testosterone level and other hormones. High testosterone levels activate the dormant cells of PCA, which act as an initial onset of adenocarcinoma [8].
One of the best therapeutic approaches for preventing the growth of PCA is the alteration of the androgen receptor (AR) [9]. A variety of biological effects are associated with AR activation. Understanding the DNA binding-dependent and independent functions of the androgen receptors (AR) in normal physiology and pathological circumstances is critical to develop targeted therapeutics for a wide range of AR-related clinical disorders. For instance, it is crucial to comprehend the ligand-binding domain (LBD) of the AR’s structure and function, as well as how it interacts with co-regulators when creating effective AR antagonists and agonists. However, it must be emphasised that aside from males with classic hypogonadism caused by organic hypothalamic-pituitary-gonadal disease, the efficacy or safety of treatment with testosterone or other AR modulators has not been shown in other populations, especially in men whose testosterone level drops with age [10].
The Prostate Specific Antigen (PSA) (also known as Ɣ-seminoprotein) is a 33-kilodalton serine-endopeptidase of the tissue kallikrein family and was recognized in prostatic extracts in the 1970s [11]. [12]Discovered PSA in human serum and [13] isolated it from prostate tissue. PSA is secreted in seminal fluid and is predominantly produced by prostatic epithelial cells (ductal and acinar) in addition to the male periurethral gland. The physiological role of PSA is to cleave semenogelins in the seminal coagulum produced for ejaculation. PSA also serves as a serum marker for PCA as it binds to proteinase inhibitors (PI’s), namely SERPINA3 and α2-macroglobulin. Normal, hyperplastic, and neoplastic prostate tissue all produce PSA. However, the highest concentration is recorded in the prostate transition zone (PTZ) of patients with prostate gland enlargement and is commonly observed in older men [14]. When physiological barriers are disrupted and basement membrane permeability is raised within the prostate, the release of PSA into the bloodstream increases. These processes include inflammation, hyper-genesis, and the presence of tumors or carcinocytes [15]. For PCA, PSA is the most widely used and divisive biomarker [16].
Medicinal plants provide a consistent and ongoing source of new therapeutic leads [17][18]. Bioactive compounds extracted from medicinal herbs have structural diversity and complexity. In addition, numerous phytochemicals that have been analyzed are reported to possess cytostatic properties and can be used to combat cancer. For example, paclitaxel (Taxol), which emanated from the Western yew tree (Taxus brevifolia), was reported as a successful antineoplastic drug for the medicament of squamous cell carcinomas (SCCs) [18][19]. In fact, most of the natural compounds reported in interventional studies are considered anticancer agents to treat malignancy [20]. Additionally, various phytochemicals have been proposed to be prophylactically beneficial in PCA therapy [21][22]. Therefore, a reverse pharmacological approach is necessary to develop an effective PCA treatment [23].
The Polynesian herbal medicinal plant Morinda citrifolia, known as noni, has a long history of therapeutic use. As per the past research report, the medicinal plant is widely known for its antagonistic effects on cancer, diabetes I&II, and viral flu, among other diseases. It is also well-known for its antibiotic, probiotic, antioxidant, antibacterial, anti-inflammatory, anticancer, and anti-diabetic activities. Each part of the plant has different phytochemical constituents with respective pharmacological activities [24]. Alizarin, damnacanthal, lauric acid, morindone, oleic acid, and scopoletin are among the most famous phytochemicals found in noni, all of which have received FDA approval and are characterized by anticancer properties [24-26], and [24].
In parallel to the rapid advancement of computer tangible and intangible components, the application of mathematical models in medicine is growing as the mathematical sciences continue to advance. Pharmacokinetics (PK)/pharmacodynamics (PD) models and innovative clinical trial designs can be formulated using computational oncology to refine drug dosing and scheduling. Treatment planning and adaptation can be enhanced by using mathematical strategies to predict tumor response, effectiveness, and toxicity. Multiscale modeling and PK/PD modeling can improve the provision of precision medicine by ensuring an optimal efficacy-toxicity balance. Oncology drug regimens can be rationally designed with dedicated models to improve potency and reduce toxicity effects [27]. Therefore, and considering the previous points, this research aimed to assess the potential antagonistic affinity of M. citrifolia phytochemicals against PCA.
Methodology
Ligands and receptor proteins
The list of phytochemicals with anticancer properties present in Morinda citrifolia was obtained from previously published scientific literature [24-26]. Six compounds were selected for the molecular docking studies. These compounds were Alizarin, Damnacanthal, Lauric acid, Morindone, Oleic acid, and Scopoletin. PubChem (3D conformer) database was used to retrieve ligands’ 3D conformers in SDF and mol2 file formats.
PCA antagonists include androgen receptors (AR) at active site chain A and prostate-specific antigens (PSA) at active site chain A (auth P) [28][29]. KEGG Database [30-32] was used to select the enzymes from the prostate cancer pathway. Then, the target proteins for prostate cancer were retrieved from the Worldwide Protein Data Bank (PDB) [33]:
- AR (Androgen receptors) active site chain A; PDB codes: 3RLJ, 5T8J, 5VO4
- PSA (Prostate-specific antigen) active site chain A (auth P); PDB codes: 2ZCH, 2ZCK, 2ZCL
Molecular-docking
The AR and PSA proteins were docked with the selected ligands (phytochemicals) using the SwissDock [34] docking software. Protein PDB files and ligands mol2 files were used in docking process. The docking results were then obtained and visualized using the UCSF Chimera [35] software. The visualizing software provides the affinity value in the form of delta G for the respective docked molecule.
Results and Discussion
Pharmacokinetics and toxicological predictions
The physicochemical, pharmacokinetic, and toxicological parameters of the selected phytochemicals were predicted using the SwissADME server [36]. The server-based tools provide essential information such as molecular weight, general properties, polarity, topological polar surface area (TPSA), the number of hydrogen bonds acceptors/donors, lipophilicity, flexibility, water solubility, pharmacokinetic properties, drug-likeness, and medicinal chemistry (Supplementary Tables 1-7).
The molecular weight of the selected phytochemicals ranged from 192.17 to 282.5 g/mol (Supplementary Table1). The fraction of CsP3 hybridization ranged between 0 and 0.92. The number of rotatable bonds was between 0 and 15. The number of hydrogen bond acceptors and donors was less than 5 in all molecules. Molar refractivity ranged between 51 and 89.94, while topological polar surface area ranged between 37.3 and 94.83 Å2 (Supplementary Table 2). All these values were within the recommended ranges for oral bioavailability according to [36], where the ranges should be from 150 to 500 for molecular weight, 40 to 130 for molar refractivity, and 20 to 130 Å2 for topological polar surface area. Additionally, all molecules fulfilled the condition of having hydrogen bond acceptors and donors less than 10 and 5, respectively. However, only lauric acid and oleic acid fulfilled the condition of CsP3 hybridization fraction (0.25 < Fraction CsP3 < 1). On the other hand, these two molecules failed to fulfill the flexibility condition (The number of rotatable bonds) since both had more than 9 rotatable bonds. Oleic acid has the highest consensus Log Po/w value (5.71), while scopoletin has the lowest (1.52) (Supplementary Table 3).
As for solubility, all the investigated phytochemicals were soluble in water except for oleic acid, which is poorly soluble (-5.41), whereas scopoletin displays a good solubility result (-2.46) (Supplementary Table 4). Calculating the projected values (log S) of molar solubility in water is based on the decimal log of the solubility. A qualitative solubility class is also available through SwissADME, and solubility can be expressed in mol/l and mg/ml. A value below -10 is considered insoluble, whereas a value above -4 is considered soluble according to SwissADME solubility measurements (log S). [36]. In fact, a soluble molecule simplifies many aspects of drug development, particularly handling and formulation. When it comes to oral administration, researchers have found that solubility plays a vital role in determining absorption [37]. When a drug is planned for non-oral means of administration, high water solubility is required to deliver an adequate amount of active ingredients, especially when administrating it at small doses [38].
In the pharmacokinetics section of the report (Supplementary Table 5), various ADME characteristics of the substance under study are assessed using specialized models. The skin permeability coefficient (Kp) is predicted by a multiple linear regression model (MLR). Thiscoefficient is linearly related to molecule size and lipophilicity (R2= 0.67) [39]. Log Kp (cm/s) measures how permeable a molecule is to the skin. The higher the -ve value of Log Kp, the less the chance of the molecules permeating the skin [36]. Among the phytochemicals mentioned above, scopoletin (-6.39 cm/s) is the least skin permeant, whereas oleic acid (-2.60 cm/s) has high skin permeability. Compounds with high skin permeability are not good candidates for oral medication but are suitable for transdermic treatments and maquillage.
It is possible to classify small molecules based on their propensity to be substrates or inhibition of key pharmacokinetic proteins [40]. Most cancer cells over-express P-gp leading to multidrug-resistant tumors [41]. Drug metabolism is influenced by the activity of CYP450 enzymes (CYPs: 1A2, 2C9, 2C19, 2D6, and 3A4), which metabolize the bulk of small molecules or phytochemicals. Computer-based methods can make a vital contribution to estimating the metabolomic properties of phytochemicals, especially during the early phase of drug discovery [42]. The studied phytochemicals have high gastrointestinal absorption. Phytochemicals such as alizarin, lauric acid, and scopoletin are blood-brain barrier permeants, whereas damnacanthal, morindone, and oleic acid are non-BBB permeants (Supplementary Table 5). All the phytonutrients mentioned above can be contemplated to target specified enzymes for their curative effects without P-glycoprotein binding. Alizarin, damnacanthal, morindone, oleic acid, and scopoletin were also CYP1A2 inhibitors. Only oleic acid was a CYP2C9 inhibitor. Alizarin, damnacanthal, and morindone were also found to be CYP3A4 inhibitors. In contrast, none of the studied phytochemicals was CYP2C19 or CYP2D6 inhibitor.
Oleic acid failed all drug-likeness filters, scopoletin failed the Muegge filter, while the rest of the studied molecules passed all drug-likeness filters [43] (Supplementary Table 6). Medicinal chemistry results (Supplementary Table 7) showed that lauric acid, oleic acid, and scopoletin have 0 alert PAIN signal, whereas damnacanthal has 1, and each of alizarin and morindone has 2.
Based on the previous results, bioavailability radar visualization (Supplementary Fig. 1) was used to predict the oral bioavailability of the investigated ligands. The results showed that all phytochemicals failed the oral bioavailability test. Alizarin, damnacanthal, morindone, and scopoletin were not orally bioavailable due to high insaturation. Oleic acid was not orally bioavailable due to high flexibility and lipophilicity, while the high flexibility of Lauric acid rendered it non-bioavailable orally. On the other hand, boiled egg visualization (Supplementary Fig. 2) showed that damnacanthal, morindone, and oleic acid have high gastrointestinal absorption since these molecules were observed more in the white zone of the egg.
Molecular docking
Molecular docking coordinates were estimated using PyRx. The grid box was set to 50 Å × 50 Å × 50 Å centered at the docking coordinates of each PDB structure (Table 1), and the exhaustiveness value was set to 8. Docking simulations were performed using Swiss Dock (blind docking). Among the six small molecules, oleic acid showed the highest binding affinity with LBD of PSA structures (2ZCH: ΔG = -7.59 kJ/mol, 2ZCK: ΔG = -8.41 kJ/mol, and 2ZCL: ΔG = -8.27 kJ/mol), while having the weakest complexes with AR (3RLJ: ΔG = -6.38 kJ/mol and 5VO4: ΔG = -6.94 kJ/mol). On the other hand, alizarin, lauric acid, and morindone showed higher affinities with LBD of AR structures (Table 2) (Figs 1-6). Oleic acid is well known for its value in traditional medicine and its potent anticancer effects [44]. Furthermore, various anticancerous effects were reported for alizarin [45], lauric acid [46], and morindone [47]. Therefore, the current computational results may encourage testing these compounds in vivo to confirm the suitability of these bioactive molecules in PCA treatment.
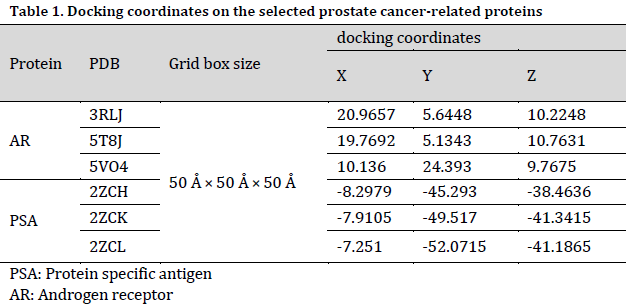
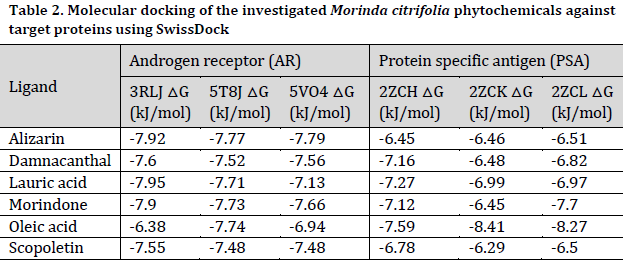
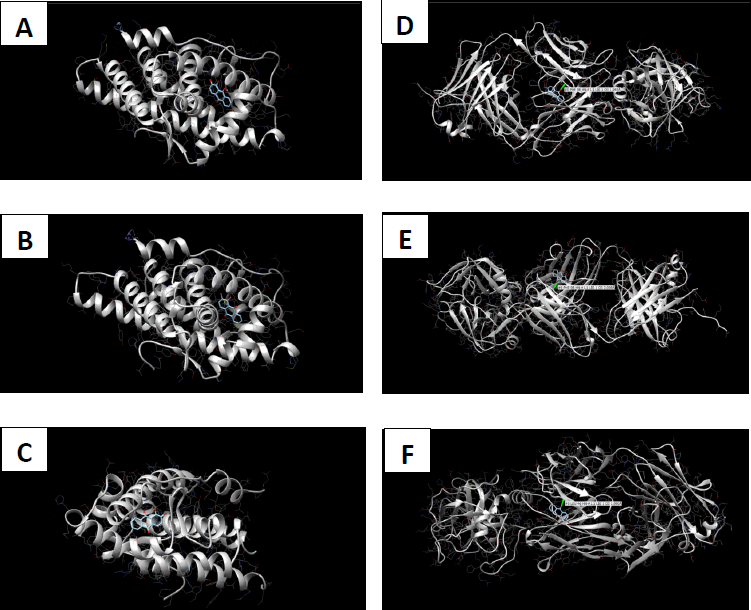
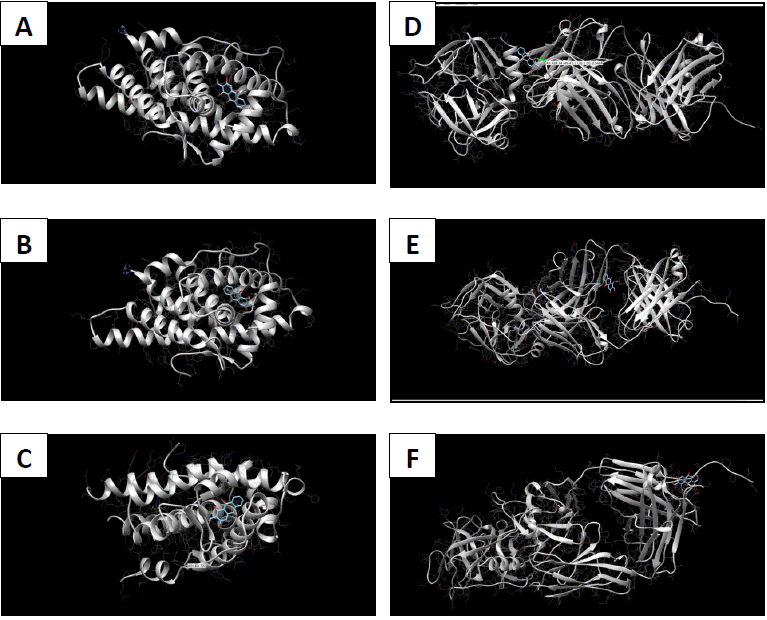
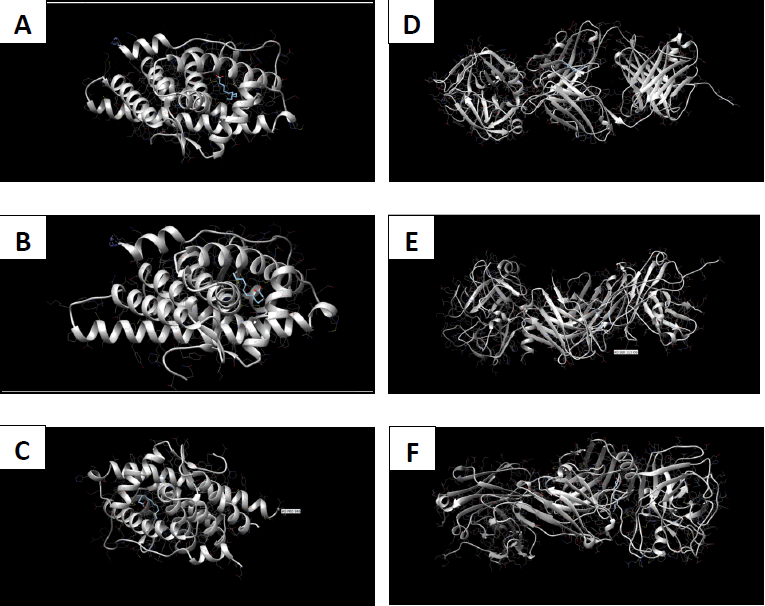
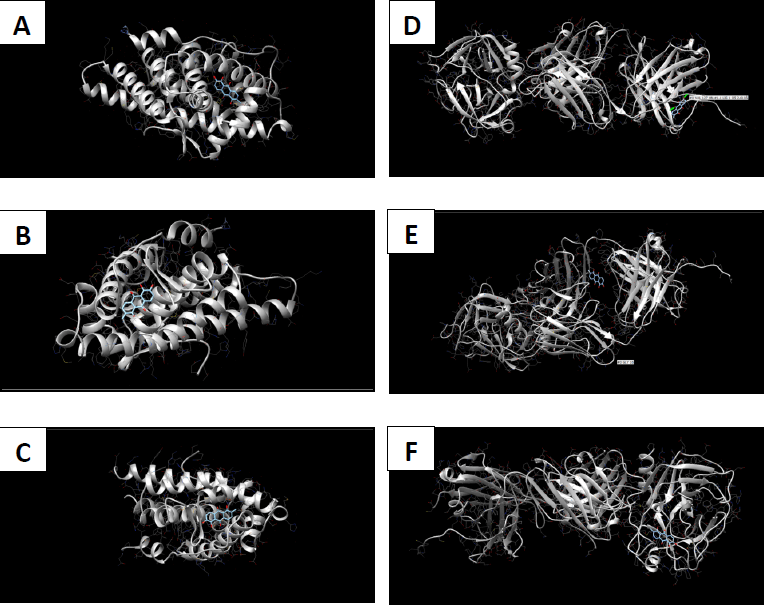
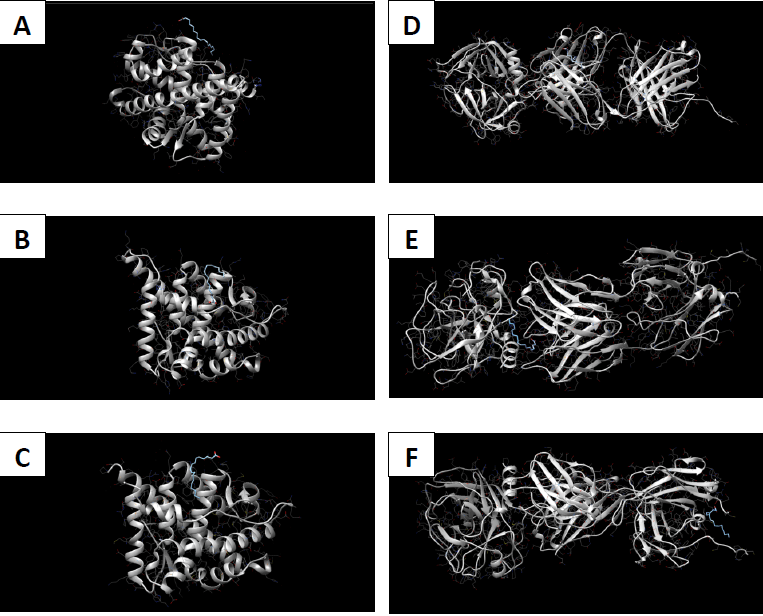
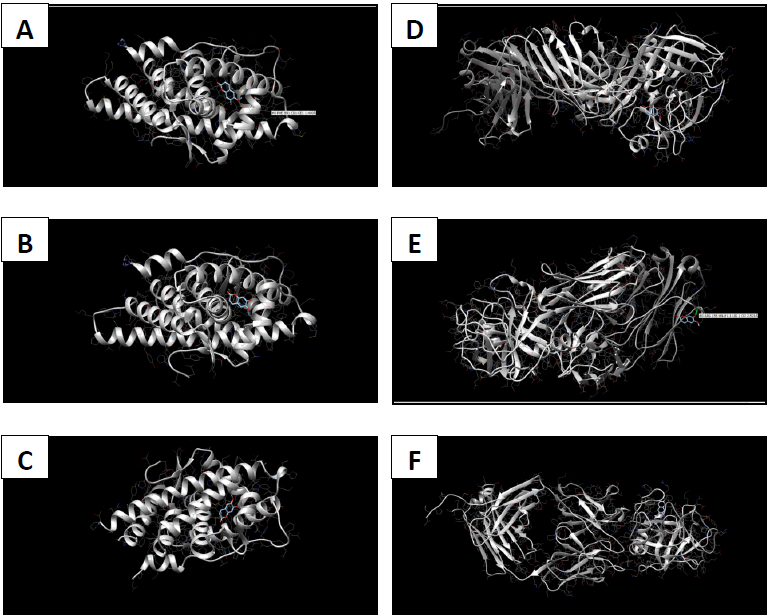
Conclusions
The study aimed to use friendly in silico studies for free ADME/T and target prediction of small bioactive molecules derived from Morinda citrifolia Linn. Most of the tested compounds exhibited suitable pharmacokinetics and drug-likeness. Damnacanthal, morindone, and oleic acid have high gastrointestinal absorption. However, all tested molecules triggered oral administration screening tests. Therefore, more research is needed to investigate better methods of administration. Molecular docking results showed that oleic acid formed more stable complexes with PSA structures, while alizarin, lauric acid, and morindone formed more stable complexes with AR structures. Due to the previous reports of anticancerous effects of these phytochemicals in a broad spectrum of cancerous cells, the current results encourage conducting in vivo tests to evaluate the effectiveness of these compounds against prostatic carcinoma.
Conflict of interest statement
The authors declared no conflict of interest.
Funding statement
The authors declared that no funding was received in relation to this manuscript.
Data availability statement The authors declared that all related data are included in the article and its supplementary material.
References
1 | RM J, Ray A, Naik D, Nath Sanyal D, Shah D. Review and research analysis of computational target methods using BioRuby and in silico screening of herbal lead compounds against pancreatic cancer using R programming. Curr. Drug Metab. 2014;15(5):535-43. DOI |
2 | Graham LS, Schweizer MT. Mismatch repair deficiency and clinical implications in prostate cancer. Prostate. 2022;82 Suppl 1:S37-S44. DOI |
3 | NaAllah A, Ayipo YO, Komolafe DI, Solihu S, Bamidele B, Alabi MA, Balogun AA, Abdulazeez AT, Mordi MN. Phytochemical screening and in silico pharmacological profiling of ethanolic extract of Aframomum melegueta for prostate carcinoma. J. Appl. Pharm. Sci. 2021;11(7):132â-45. DOI |
4 | Ashrafizadeh M, Paskeh MDA, Mirzaei S, Gholami MH, Zarrabi A, Hashemi F, Hushmandi K, Hashemi M, Nabavi N, Crea F, Ren J, Klionsky DJ, Kumar AP, Wang Y. Targeting autophagy in prostate cancer: preclinical and clinical evidence for therapeutic response. J. Exp. Clin. Cancer. Res. 2022;41(1):105. DOI |
5 | International Agency for Research on Cancer. Estimated number of new cases in 2020, worldwide, for both sexes, and all ages (excl. NMSC). WHO, 2020. Link |
6 | SEER Cancer Stat Facts: Prostate Cancer. National Cancer Institute. Bethesda, MD. 2022. Link |
7 | Key Statistics for Prostate Cancer; American Cancer Society: Atlanta, GA, USA. ACS. 2018 |
8 | Fleshner N, Bagnell PS, Klotz L, Venkateswaran V. Dietary fat and prostate cancer. J. Urol. 2004;171(2):S19-24. DOI |
9 | Ikwu FA, Shallangwa GA, Mamza PA. QSAR, QSTR, and molecular docking studies of the anti-proliferative activity of phenylpiperazine derivatives against DU145 prostate cancer cell lines. Beni-Suef Univ. J. Basic Appl. Sci. 2020;9(1). DOI |
10 | Davey RA, Grossmann M. Androgen Receptor Structure, Function and Biology: From Bench to Bedside. Clin. Biochem. Rev. 2016;37(1):3-15. |
11 | Ablin RJ, Soanes WA, Bronson P, Witebsky E. Precipitating antigens of the normal human prostate. J Reprod. Fertil. 1970;22(3):573-4. DOI |
12 | Wang MC, Valenzuela LA, Murphy GP, Chu TM. Purification of a human prostate specific antigen. Invest. Urol. 1979;17(2):159-63. |
13 | Papsidero LD, Wang MC, Valenzuela LA, Murphy GP, Chu TM. A prostate antigen in sera of prostatic cancer patients. Cancer Res. 1980;40(7):2428-32. |
14 | Stamey TA, Yang N, Hay AR, McNeal JE, Freiha FS, Redwine E. Prostate-specific antigen as a serum marker for adenocarcinoma of the prostate. N. Engl. J. Med. 1987;317(15):909-16. DOI |
15 | Tchetgen MB, Oesterling JE. The role of prostate-specific antigen in the evaluation of benign prostatic hyperplasia. Urol. Clin. North Am. 1995;22(2):333-44. DOI |
16 | Pentyala S, Whyard T, Pentyala S, Muller J, Pfail J, Parmar S, Helguero CG, Khan S. Prostate cancer markers: An update. Biomed. Rep. 2016;4(3):263-268. DOI |
17 | Cragg GM, Newman DJ. Natural products: a continuing source of novel drug leads. Biochim. Biophys. Acta. 2013;1830(6):3670-95. DOI |
18 | Zulkipli IN, David SR, Rajabalaya R, Idris A. Medicinal Plants: A Potential Source of Compounds for Targeting Cell Division. Drug Target Insights. 2015;9:9-19. DOI |
19 | Weaver BA. How Taxol/paclitaxel kills cancer cells. Mol. Biol. Cell. 2014 Sep 15;25(18):2677-81. DOI |
20 | Butler MS, Robertson AA, Cooper MA. Natural product and natural product derived drugs in clinical trials. Nat. Prod. Rep. 2014;31(11):1612-61. DOI |
21 | Reed D, Raina K, Agarwal R. Nutraceuticals in prostate cancer therapeutic strategies and their neo-adjuvant use in diverse populations. NPJ Precis. Oncol. 2018;2:15. DOI |
22 | Wang Z, Fan J, Liu M, Yeung S, Chang A, Chow MS, Pon D, Huang Y. Nutraceuticals for prostate cancer chemoprevention: from molecular mechanisms to clinical application. Expert Opin. Investig. Drugs. 2013;22(12):1613-26. DOI |
23 | Surh YJ. Reverse pharmacology applicable for botanical drug development – inspiration from the legacy of traditional wisdom. J. Tradit. Complement. Med. 2011;1(1):5-7. DOI |
24 | Patil P. Morinda citrifolia L.(Noni)–Its ethnobotanical knowledge, phytochemical studies, pharmacological aspects, and future prospects. Int. J. Nov. Res. Dev. 2022;7(3):331-52. DOI |
25 | Anekpankul T, Goto M, Sasaki M, Pavasant P, Shotipruk A. Extraction of anticancer damnacanthal from roots of Morinda citrifolia by subcritical water. Sep. Purif. Technol. 2007;55(3):343-9. DOI |
26 | Saminathan M, Rai RB, Dhama K, Tiwari R, Chakraborty S, Amarpal RG, Kannan K. Systematic review on anticancer potential and other health beneficial pharmacological activities of novel medicinal plant Morinda citrifolia (Noni). Int. J. Pharmacol. 2013;9(8):462-92. DOI |
27 | Barbolosi D, Ciccolini J, Lacarelle B, Barlési F, André N. Computational oncology—mathematical modelling of drug regimens for precision medicine. Nature reviews Clinical oncology. 2016;13(4):242-54. DOI |
28 | Feng Q, He B. Androgen Receptor Signaling in the Development of Castration-Resistant Prostate Cancer. Front. Oncol. 2019;9:858. DOI |
29 | Balk SP, Ko YJ, Bubley GJ. Biology of prostate-specific antigen. J. Clin. Oncol. 2003;21(2):383-91. DOI |
30 | Kanehisa M, Goto S. KEGG: kyoto encyclopedia of genes and genomes. Nucleic Acids Res. 2000;28(1):27-30. DOI |
31 | Kanehisa M. Toward understanding the origin and evolution of cellular organisms. Protein Sci. 2019;28(11):1947-51. DOI |
32 | Kanehisa M, Furumichi M, Sato Y, Ishiguro-Watanabe M, Tanabe M. KEGG: integrating viruses and cellular organisms. Nucleic Acids Res. 2021;49(D1):D545-51. DOI |
33 | Berman H, Henrick K, Nakamura H. Announcing the worldwide protein data bank. Nature Nat. Struct. Mol. Biol. 2003;10(12):980. DOI |
34 | Grosdidier A, Zoete V, Michielin O. SwissDock, a protein-small molecule docking web service based on EADock DSS. Nucleic Acids Res. 2011;39(suppl_2):W270-7. DOI |
35 | Pettersen EF, Goddard TD, Huang CC, Couch GS, Greenblatt DM, Meng EC, Ferrin TE. UCSF Chimera—a visualization system for exploratory research and analysis. J. Comput. Chem. 2004;25(13):1605-12. DOI |
36 | Daina A, Michielin O, Zoete V. SwissADME: a free web tool to evaluate pharmacokinetics, drug-likeness and medicinal chemistry friendliness of small molecules. Sci. Rep. 2017 Mar 3;7:42717. DOI |
37 | Ottaviani G, Gosling DJ, Patissier C, Rodde S, Zhou L, Faller B. What is modulating solubility in simulated intestinal fluids? Eur. J. Pharm. Sci. 2010;41(3-4):452-7. DOI |
38 | Savjani KT, Gajjar AK, Savjani JK. Drug solubility: importance and enhancement techniques. Int. Sch. Res. Notices. 2012:195727. DOI |
39 | Potts RO, Guy RH. Predicting skin permeability. Pharm. Res. 1992;9(5):663-9. DOI |
40 | Daina A, Zoete V. A BOILED-Egg To Predict Gastrointestinal Absorption and Brain Penetration of Small Molecules. ChemMedChem. 2016;11(11):1117-21. DOI |
41 | Mahanthesh M, Ranjith D, Yaligar R, Jyothi R, Narappa G, Ravi M. Swiss ADME prediction of phytochemicals present in Butea monosperma (Lam.) Taub. J. Pharmacogn. Phytochem.. 2020;9(3):1799-809. |
42 | Plonka W, Stork C, Šícho M, Kirchmair J. CYPlebrity: Machine learning models for the prediction of inhibitors of cytochrome P450 enzymes. Bioorg. Med. Chem. 2021;46:116388. DOI |
43 | Pathania S, Singh PK. Analyzing FDA-approved drugs for compliance of pharmacokinetic principles: should there be a critical screening parameter in drug designing protocols?. Expert Opin. Drug Metab. Toxicol. 2021;17(4):351-4. DOI |
44 | Jiang L, Wang W, He Q, Wu Y, Lu Z, Sun J, Liu Z, Shao Y, Wang A. Oleic acid induces apoptosis and autophagy in the treatment of Tongue Squamous cell carcinomas. Sci. Rep. 2017;7(1). DOI |
45 | Xu Z, Hou Y, Zou C, Liang H, Mu J, Jiao X, Zhu Y, Su L, Liu M, Chen X, Qian C. Alizarin, a nature compound, inhibits the growth of pancreatic cancer cells by abrogating NF-κB activation. Int. J. Biol. Sci. 2022;18(7):2759. DOI |
46 | Verma P, Ghosh A, Ray M, Sarkar S. Lauric Acid Modulates Cancer-Associated microRNA Expression and Inhibits the Growth of the Cancer Cell. Anticancer Agents Med. Chem. 2020;20(7):834-44. DOI |
47 | Chee CW, Zamakshshari NH, Lee VS, Abdullah I, Othman R, Lee YK, Mohd Hashim N, Nor Rashid N. Morindone from Morinda citrifolia as a potential antiproliferative agent against colorectal cancer cell lines. PloS one. 2022;17(7):e0270970. DOI |
Cite this article:
Patil, P., Shah, D. N. In silico pharmacokinetic analysis of Morinda citrifolia phytochemicals and their potential antagonistic effect on prostatic carcinoma proteins. DYSONA – Life Science, 2022;3(2): 83-96. doi: 10.30493/dls.2022.351879