Raed S. Shehata 1*
1, Department of Agriculture, Ministry of Agriculture and land reclamation, Damanhour, Egypt
E-mail:
Raedsalem8882001@gmail.com
Received: 31/07/2024
Acceptance: 15/08/2024
Available Online: 16/08/2024
Published: 01/01/2025
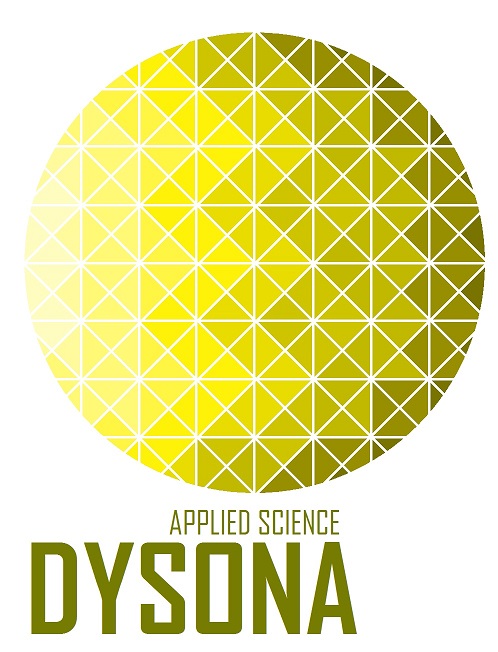
Manuscript link
http://dx.doi.org/10.30493/DAS.2024.470973
Abstract
Proline is an aliphatic, nonpolar amino acid that serves as a vital osmoregulator in plants that are subjected to abiotic stresses, including heat, salinity, and drought. Proline is endogenously produced in plant to enhance its ability to withstand adverse environmental conditions. Hence, the accumulation of proline in plant tissue is commonly recognized as a characteristic plant response to external stresses. Multiple studies have shown that the exogenous administration of proline at low concentrations improves stress tolerance, leading to an increase in fruit yield and boosting their quality. This review examines the influence of proline applications on the quality of fruit and the processes through which proline improves fruit quality, as documented in the literature.
Keywords: Proline, Fruit quality, Oxidative stress, Postharvest, Pre-harvest
Introduction
Proline, an amino acid, is essential for facilitating plants’ ability to adjust to environmental stresses [1][2]. Proline is an aliphatic, nonpolar amino acid that has multiple roles in biological systems. Osmotic adjustment plays a crucial role in this process [3]. The accumulation of this substance is a typical plant reaction to different environmental stresses, serving as an organic osmolyte that aids plants in withstanding osmotic stresses. The presence of osmolytes, such as proline, has been strongly associated with a plant’s capacity to endure and adjust to difficult environmental conditions. This phenomenon is commonly observed in plants that are subjected to stress [4][5][6].
Proline is a highly important and thoroughly researched osmolyte in terms of its ability to improve plant’s tolerance to abiotic stresses. Furthermore, the external administration of proline has been demonstrated to enhance fruit yield under abiotic stress conditions [7], promoting fruit formation and growth, as well as improving the overall quality of the fruit [8].
This study delves into proline synthesis in plant and investigates the effects of exogenous proline application on the quality of fruits, both pre- and post-harvest.
Proline synthesis
Proline is synthesized by plants using glutamate and ornithine as precursors. Many pathways of proline production in various life domains were previously investigated [9] (Fig. 1). The accumulation of proline during times of stress is affected by both the promotion of its production and the suppression of its breakdown [10]. Plant cells utilize the cytosol to produce proline for fundamental cellular functions. Nevertheless, once stress conditions are identified, the synthesis of proline relocates to the chloroplasts [11]. Pyroline-5-carboxylate synthetase (P5CS) is a dual-function enzyme that facilitates the major pathway of proline synthesis in higher plants and animals. Glutamate serves as the substrate for proline production in this step. Ornithine, derived from the initial steps of the arginine biosynthesis route, is utilized in an alternate pathway for the production of proline. Plants prioritize the glutamate pathway for proline synthesis during osmotic stress [10].
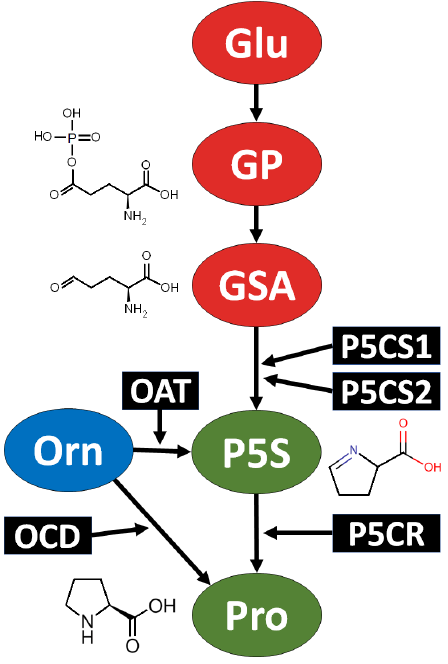
Proline synthesis from glutamate
The initial discoveries revealed that bacteria have the ability to produce proline from glutamate [12]. This pathway is essential for plants during osmotic stress or nitrogen deficiency [13]. The enzymes pyrroline-5-carboxylate synthetase (P5CS) and pyrroline-5-carboxylate reductase (P5CR) are mainly responsible for converting glutamate into proline by using pyrroline-5-carboxylate (P5C) as an intermediate [14][15]. The P5CR enzyme in plants is encoded by a solitary gene, whereas the P5CS enzyme is represented by two separate genes, namely P5CS1 and P5CS2 [16]. P5CS1 is predominantly expressed in the shoots and roots in reaction to stress or when supplemented with abscisic acid (ABA). The enzyme P5CS2 is expressed at significant levels in proliferative cells, endosperm, and inflorescences [17].
The first step in the production of proline from glutamate is the phosphorylation of glutamate to become γ-glutamyl phosphate (GP). GP is converted into an intermediate compound known as glutamic semialdehyde (GSA), which is then converted by the P5CS enzyme complex into Δ1-pyrroline-5-carboxlyate (P5C). Ultimately, the P5CR enzyme in both prokaryotes and eukaryotes converts P5C into l-proline. P5CS is an essential regulatory enzyme that governs the production of proline in plants and acts as a gatekeeper of proline synthesis [18]. Transcriptional regulation and feedback inhibition exert control over this pathway [19] and [20]. In that regard, light [21] and nitric oxide [22] were found to have a positive influence on P5CS activity, whereas brassinosteroids have a detrimental impact [23].
Proline synthesis from ornithine
During supra-optimal nitrogen conditions and the seed development stage, L-proline is produced through a different pathway that utilizes ornithine as the precursor [16][24]. In this pathway, the amino group of ornithine undergoes transamination through the intermediate product pyrroline-2-carboxylate. This process is facilitated by the enzyme ornithine-Δ-aminotransferase (OAT), resulting in the production of P5C [25][26]. Additionally, the enzyme ornithine cyclodeaminase (OCD) can directly convert ornithine into l-proline.
Applications of proline in enhancing fruit quality
The exogenous application of proline was investigated in literature to explore its potential in enhancing fruit production in terms of quantity and quality (Table 1).
Studies on peach trees have demonstrated that the incorporation of proline and potassium silicate significantly enhances the fruit’s quality. This is achieved by augmenting the quantities of anthocyanin and carotenoid in the skin, while also elevating the levels of total soluble solids (TSS) and vitamin C in the fruit. According to these data, it seems that using proline might greatly improve the visual attractiveness and nutritional value of fruits, making them more appealing to consumers [7]. The application of proline, especially in combination with fruit bagging, led to improved skin and aril pigmentation, greater levels of anthocyanin, and higher total soluble solids (TSS) content in pomegranates. It can be deduced that proline enhances not just the visual appeal of fruits, but also their taste and nutritional value [8].
The positive impacts of proline extend beyond enhancing fruit quality to enhancing productivity under unfavorable conditions. For instance, utilizing both proline and magnetized water enhanced the growth of pomegranate trees, increased chlorophyll levels, and improved the nutritional health of the trees when they were exposed to limited water availability and high salinity. As a consequence, there was an increase in crop production and improved fruit characteristics, such as a decrease in fruit cracking and an enhancement in the levels of total soluble solids and vitamin C content [27]. This illustrates that proline has the capacity to help alleviate the detrimental impacts of environmental stress on fruit yield.
Additional research conducted on various fruit crops have consistently demonstrated that proline has a positive impact on enhancing fruit quality in diverse circumstances. Applying L-proline to citrus trees through foliar spraying led to an increment in fruit weight, diameter, and total soluble solids (TSS), suggesting its capacity to enhance both the size and flavor of the fruit [28]. Similarly, it was shown that the use of proline on young Japanese pear trees led to a rise in sugar levels and enhanced nitrogen metabolism, resulting in higher fruit quality [29]. Studies have also shown that the use of proline, together with additional treatments like tryptophan, can enhance the yield and quality of fruit in pomegranate orchards, while reducing the occurrence of cracked fruits [30].
The efficacy of proline is also shown in other varieties of crops. Applying proline as a spray directly on the leaves of olive trees helped alleviate the detrimental impact of salinity on their growth and photosynthetic activity. This, in turn, resulted in enhanced fruit production and increased fruit quality. The objective was achieved by maintaining a higher amount of water in the leaves and enhancing the activity of antioxidant enzymes, which safeguard the plant from oxidative harm during periods of stress [31][32].
Proline treatment has been found to increase osmoprotectant mechanisms in pomegranate trees, enhancing the plant’s nutritional status and osmoregulatory functions when exposed to salt stress. The study demonstrated that the combination of proline and humic acid led to a substantial rise in leaf proline, total carbohydrates, and key nutrient levels. This increase ultimately resulted in enhanced fruit output and quality [33]. Furthermore, in sweet cherry orchards, the absorption and distribution of proline applied to the leaves were shown by its movement to different plant components, including the fruit. The application of this treatment resulted in enhancements in the characteristics of fruit quality. However, it also accelerated the ripening process of the fruit, leading to smaller yet more mature fruits [34].
The application of proline to grapevines resulted in improved yield components, including increased cluster weight and a higher number of clusters per vine. Furthermore, essential measures of quality, such as the amount of sugars, anthocyanin content, and total soluble solids (TSS), were also enhanced. These findings indicate that proline has the capacity to significantly enhance both the quantity and quality of grapes in viticulture [35]. Moreover, studies have shown that the use of proline can enhance the plant’s antioxidative defense mechanism. An experiment was conducted on mango trees to investigate the effects of applying proline and algal extracts. The results showed that this combination led to an enhancement in the activity of crucial antioxidant enzymes. This not only enhanced the plant’s resilience to stress, but also enhanced the quality of its fruits during periods of abiotic stress [36].
Postharvest application of proline and L-cysteine through emersion treatment effectively reduced internal browning and titratable acidity in peach fruit, while maintaining its firmness [37]. Additionally, the exogenous application of proline reduced chilling injury and electrolyte leakage in treated grapes during cold storage [38]. These findings show that proline direct application on fruit can have a positive influence on storability and the overall postharvest performance of treated fruit. Therefore, exploring this effect on other fruit crops can be an interesting field of research.
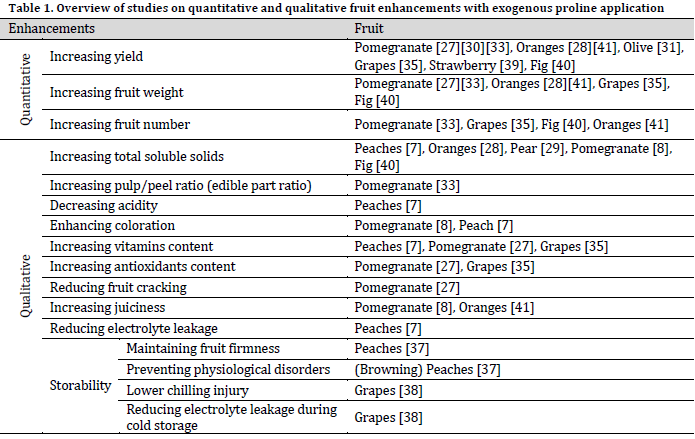
Proline mode of action in enhancing fruit quality
Proline and osmoregulation
In conditions of stress including heat, salinity and water stress, osmoregulation is crucial for preserving cellular integrity. Raising proline concentrations lowers plant cells’ osmotic potential, which preserves cell turgor and facilitates water absorption. Due to the rise in fruit size and weight, this osmotic adjustment is essential for maintaining cellular function and promoting development in situations with limited water availability, which increases yield [42]. Proline has the ability to increase the stability of proteins and cellular structures, protecting them from harm caused by dehydration and high salt concentrations [2]. Proline is a potent solute that helps cells regulate their ion levels, especially when they are under salt stress. Fruit quality overall and firmness are enhanced by this regulation [25].
Proline and antioxidant capacity
Proline is crucial for safeguarding plants from oxidative stress induced by environmental stimuli (Fig. 2). It directly scavenges reactive oxygen species (ROS), including superoxide radicals and hydrogen peroxide, to prevent oxidative damage to biological components such proteins, lipids, and nucleic acids [43]. Proline also increases the efficiency of vital antioxidant enzymes that are essential for lowering oxidative stress in plant cells and neutralizing damaging reactive oxygen species (ROS), such as catalase (CAT), peroxidases (POD), and superoxide dismutase (SOD) [11]. The structural integrity of cells and the firmness, color, and overall quality of fruits are dependent on the improved antioxidant defense system. Furthermore, proline has a vital role in maintaining the balance of cellular redox, which is necessary for optimizing metabolic processes that enhance the development of fruits with a prolonged shelf life and high nutritional content [44].
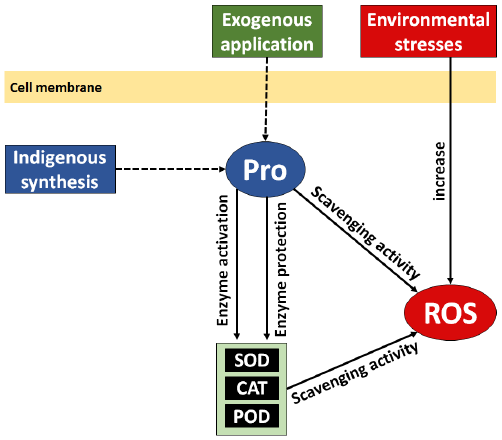
Proline and photosynthesis
Since photosynthesis is essential to plant development and productivity, it is also essential for sustaining high-quality fruit under unfavorable conditions. Proline is essential for protecting and strengthening the photosynthetic apparatus, particularly in adverse environmental conditions. It results in steady and efficient photosynthetic activity by maintaining the structural integrity of chloroplasts and preventing the breakdown of the photosystem complex [45]. Additionally, proline affects stomatal conductance, which lowers water loss and improves CO2 absorption, enabling photosynthesis to proceed even in stressful situations [46]. Furthermore, there is evidence that proline administration is associated with an increase in chlorophyll content. This raises the efficiency of photosynthetic activities by enhancing light absorption and utilization [47]. All of these factors interact in concert to improve photosynthetic efficiency, which in effect leads to increased fruit productivity. This is evident in larger fruit sizes, higher yields, and improved quality attributes like taste, color, and nutritional content.
Conclusions and future aspects
Utilizing proline, a naturally occurring amino acid, to enhance fruit quality is an innovative method for growing nutritious food without any residual substances, so protecting humanity from potential illnesses. Proline helps stress alleviating mechanisms in fruit trees resulting in an increase in production quantity and fruit quality. Hence, further investigation is warranted to validate the optimal dosages of proline, the ideal timing of pre-harvest application, and the frequency at which proline should be administered to fruit trees in order to attain the utmost fruit yield and quality. Furthermore, it is crucial to investigate the potential of proline as a postharvest treatment during fruit storage due to its highly promising ability to protect against storage stresses, particularly chilling injury.
Acknowledgements
I am deeply grateful to assistant lecturer: Sara Reda Alaswad (Damanhour University) for her dedicated time, expertise and helping me to finish this review.
References
- Saibi W, Feki K, Ben Mahmoud R, Brini F. Durum wheat dehydrin (DHN-5) confers salinity tolerance to transgenic Arabidopsis plants through the regulation of proline metabolism and ROS scavenging system. Planta. 2015;242(5):1187–94. DOI
- Szabados L, Savouré A. Proline: a multifunctional amino acid. Trends Plant Sci. 2010;15(2):89–97. DOI
- Hmidi D, Abdelly C, Athar HU, Ashraf M, Messedi D. Effect of salinity on osmotic adjustment, proline accumulation and possible role of ornithine-δ-aminotransferase in proline biosynthesis in Cakile maritima. Physiol. Mol. Biol. Plants. 2018;24:1017–33. DOI
- Errabii T, Gandanou CB, Essalmani H, Abrini J, Idamor M, Senhaji NS. Effect of NaCl and mannitol induced stress on sugarcane (Saccharum sp.) callus cultures. Acta Physiol. Plant. 2007;29:95–102. DOI
- Slama I, Ghnaya T, Savouŕe A, Abdelly C. Combined effects of long-term salinity and soil drying on growth, water relations, nutrient status and proline accumulation of Sesuvium portulacastrum. C. R. Biol. 2008;331:442–51. DOI
- Ahire ML, Walunj PR, Kavi Kishor PB, Nikam TD. Effect of sodium chloride induced stress on growth, proline, glycine betaine accumulation, antioxidative defense and bacoside a content in in vitro regenerated shoots of Bacopa monnieri (L.) Pennell. Acta Physiol. Plant. 2013;35:1943–53. DOI
- Shehata RS, Farag KM. Effect of Potassium Silicate, Glycine Betaine and Proline on Fruit Quality of Peaches in Newly Reclaimed Land Exposed to Heat Stress. Hortic. Sci. 2024;41(2):42-9. DOI
- Farag KM, Shehata RS. Effect of proline and fruit bagging on the coloration of Aril and peel of “Wonderful” pomegranates. Hortic. Int. J. 2023;7(1):21-6. DOI
- Fichman Y, Gerdes SY, Kovács H, Szabados L, Zilberstein A, Csonka LN. Evolution of proline biosynthesis: enzymology, bioinformatics, genetics, and transcriptional regulation. Biol. Rev. 2015;90:1065–99. DOI
- Ben Rejeb K, Abdelly C, Savouré A. How reactive oxygen species and proline face stress together. Plant Physiol. Biochem.2014;80:278–84. DOI
- Székely G, Abraham E, Cselo A, Rigo G, Zsigmond L, Csiszar J, Ayaydin F, Strizhov N, Jasik J, Schmelzer E, Koncz C, Szabados L. Duplicated P5CS genes of Arabidopsis play distinct roles in stress regulation and developmental control of proline biosynthesis. Plant J. 2008;53:11–28. DOI
- Leisinger T. Biosynthesis of proline in Escherichia coli and Salmonella typhimurium. In: Cellular and molecular biology. American Society for Microbiology, Washington, DC. 1987;345–51.
- Trovato M, Mattioli R, Costantino P. Multiple roles of proline in plant stress tolerance and development. Rend Lincei-Sci Fis. 2008;19:325–46. DOI
- Verslues PE, Sharma S. Proline metabolism and its implications for plant-environment interaction. The Arabidopsis Book. American Society of Plant Biologists. 2010;8:e0140. DOI
- Sekhar PN, Amrutha NR, Sangam S, Verma DPS, Kavi Kishor PB. Biochemical characterization, homology modeling and docking studies of ornithine δ-aminotransferase an important enzyme in proline biosynthesis of plants. J Mol. Graph. Model. 2007;26:709–19. DOI
- Armengaud P, Thiery L, Buhot N, Ghislaine GDM, Savouré A. Transcriptional regulation of proline biosynthesis in Medicago truncatula reveals developmental and environmental specific features. Physiol. Plant. 2004;120:442–50. DOI
- Kavi Kishor PB, Sreenivasulu N. Is proline accumulation per se correlated with stress tolerance or is proline homeostasis a more critical issue? Plant Cell Environ. 2014;37:300–11. DOI
- Kavi Kishor PB, Hong Z, Miao GH, Hu CAA, Verma DPS. Overexpression of Δ1-pyrroline-5-carboxylate synthase increases proline production and confers osmotolerance in transgenic plants. Plant Physiol. 1995;108:1387–94. DOI
- Yoshiba Y, Kiyosue T, Katagiri T, Ueda H, Mizoguchi T, Yamaguchi-Shinozaki K, Wada K, Harada Y, Shinozaki K. Correlation between the induction of a gene for delta(1)-pyrroline-5-carboxylate synthetase and the accumulation of proline in Arabidopsis thaliana under osmotic stress. Plant J. 1995;7:751–60. DOI
- Zhang M, Huang H, Dai S. Isolation and expression analysis of proline metabolism-related genes in Chrysanthemum lavandulifolium. Gene. 2014;537:203–13. DOI
- Hayashi F, Ichino T, Osanai M, Wada K. Oscillation and regulation of proline content by P5CS and ProDH gene expressions in the light/dark cycles in Arabidopsis thaliana L. Plant Cell Physiol. 2000;41:1096–101. DOI
- Zhao MG, Chen L, Zhang LL, Zhang WH. Nitric reductase-dependent nitric oxide production is involved in cold acclimation and freezing tolerance in Arabidopsis. Plant Physiol. 2009;151:755–67. DOI
- Ábrahám E, Rigó G, Székely G, Nagy R, Koncz C, Szabados L. Light-dependent induction of proline biosynthesis by abscisic acid and salt stress is inhibited by brassinosteroid in Arabidopsis. Plant Mol. Biol. 2003;51:363–72. DOI
- Roosens NHCJ, Thu TT, Iskandar HM, Jacobs M. Isolation of the Ornithine-δ-Aminotransferase cDNA and effect of salt stress on its expression in Arabidopsis thaliana. Plant Physiol. 1998;117:263–71. DOI
- Verbruggen N, Hermans C. Proline accumulation in plants: a review. Amino Acids. 2008;35:753–9. DOI
- Delauney AJ, Verma DPS. Cloning of ornithine δ-aminotransferase cDNA from Vigna aconitifolia by trans-complementation in Escherichia coli and regulation of proline biosynthesis. Plant J. 1993;4:215–23.
- Okba SK, Mazrou Y, Mikhael GB, Farag MEH, Alam-Eldein SM. Magnetized Water and Proline to Boost the Growth, Productivity and Fruit Quality of ‘Taifi’ Pomegranate Subjected to Deficit Irrigation in Saline Clay Soils of Semi-Arid Egypt. Horticulturae. 2022;8:564. DOI
- Caronia A, Gugliuzza G, Inglese P. Influence of L-proline on Citrus sinensis (L.) [‘New Hall’and’Tarocco Scire’] fruit quality. In XI International Symposium on Plant Bioregulators in Fruit Production. 2009;884:423-6. DOI
- Takeuchi M, Arakawa C, Kuwahara Y, Gemma H. Effects of l-proline foliar application on the quality of ‘kosui’ japanese pear. In X International Pear Symposium. 2008;800:549-4. DOI
- El Sayed OM, El Gammal OHM, Salama ASM. Effect of proline and tryptophan amino acids on yield and fruit quality of Manfalouty pomegranate variety. Sci. Hortic. 2014;169. DOI
- El-Sayed OM, El-Gammal OHM, Salama ASM. Effect of ascorbic acid, proline and jasmonic acid foliar spraying on fruit set and yield of Manzanillo olive trees under salt stress. Sci. Hortic. 2014;176:32-7. DOI
- Ben Ahmed C, Ben Rouina B, Sensoy S, Boukhriss M, Ben Abdullah F. Exogenous proline effects on photosynthetic performance and antioxidant defense system of young olive tree. J. Agric. Food Chem. 2010;58(7):4216–22. DOI
- Rashedy AA, Abd-elnafea MH, Khedr EH. Co-application of proline or calcium and humic acid enhances productivity of salt stressed pomegranate by improving nutritional status and osmoregulation mechanisms. Sci Rep. 2022;12:14285. DOI
- Hölzel N, Close DC, Bound SA, Quin PR, Visentin DC, Swarts ND. Uptake and Translocation of Foliar-Applied L-Proline in Sweet Cherry (Prunus avium L.).Agronomy. 2023;13(4):958. DOI
- Mustafa S A, Al-Atrushy S M. Effect of Tipping and Foliar Application of Proline and Botminn Plus on Yield and Quality of Grapevine (Vitis vinifera L.) Cv. Khoshnaw. In IOPConference Series: Earth and Environmental Science. IOP Publishing. 2023;1158(4):042071. DOI
- Da Cunha JG, Cavalcante ÍHL, da Silva MA, Amariz RA, Carmo RN, Lobo JT. Proline and algal extract to alleviate the abiotic stress in mango ‘Tommy Atkins’ in the tropical semiarid. Erwerbs-Obstbau. 2022;64:115–26.DOI
- Gohari G, Molaei S, Kheiry A, Ghafouri M, Razavi F, Lorenzo JM, Juárez-Maldonado A. Exogenous Application of Proline and L-Cysteine Alleviates Internal Browning and Maintains Eating Quality of Cold Stored Flat ‘Maleki’ Peach Fruits. Horticulturae. 2021;7(11):469. DOI
- Mohammadrezakhani S, Pakkish Z. Reduction of chilling injury and peroxide hydrogen accumulation in Thompson grape (Vitis vinifera L.) fruit by proline and ascorbic acid during storage. J. Plant Physiol. Breed. 2015;5(1).
- Celiktopuz E, Kapur B, Sarıdas MA, Güney Oİ, Aksoy F. Yield, quality and physiological variation of strawberry in response to irrigation regimes and exogenous proline with a cost benefit analysis. Plant Physiol. Biochem. 2023;195:238-46. DOI
- MM Abd El-Azeem, M., R Beheiry, H., AA Mohamed, I., GA Abdel-Samad, A., AZ Hussein, H., EM Mahmoud, A. and M Rady, M., 2023. Effect of exogenous CPPU, proline, or potassium on late yield and fruit quality of fig (Ficus carica L.) trees. Fayoum J. Agr. Res. Dev. 37(4):832-44. DOI
- Sanaa MM, Abd El-Rahman GF. Effects of Exogenous Application of Glycine Betaine and Proline on Productivity of Valencia Orange Trees Grown in a Saline Soil. Hortic. Res. J. 2023;1(2):171-84 DOI
- Ashraf M, Foolad MR. Roles of glycine betaine and proline in improving plant abiotic stress resistance. Environ. Exp. Bot. 2007;59(2),206-16. DOI
- Sengupta D, Majumder AL. Osmolyte Regulation in Abiotic Stress. In Handbook of Plant and Crop Stress. CRC Press. 2010:349-67.
- Yoshiba Y, Kiyosue T, Nakashima K, Yamaguchi-Shinozaki K, Shinozaki K. Regulation of levels of proline as an osmolyte in plants under water stress. Plant Cell Physiol. 1997;38(10):1095-102. DOI
- Hayat S, Hayat Q, Alyemeni MN, Wani AS, Pichtel, J, Ahmad A. Role of proline under changing environments: A review. Plant Signaling Behav. 2012; 7(11):1456-66. DOI
- Nakashima K, Yamaguchi-Shinozaki K, Shinozaki K. Molecular responses to drought and cold stress. Curr. Opin. Biotechnol. 1996;9(2),161-7. DOI
- Kishor PK, Sangam S, Amrutha RN, Laxmi PS, Naidu KR, Rao KS, Rao S, Reddy KJ, Theriappan P, Sreenivasulu N. Regulation of proline biosynthesis, degradation, uptake and transport in higher plants: its implications in plant growth and abiotic stress tolerance. Curr. Sci. 2005:424-38.
Cite this article:
Shehata, R. S. Proline in action: Enhancing fruit quality. DYSONA – Applied Science, 2025;6(1): 8-15. doi: 10.30493/das.2024.470973