Nik M. H. Nik Abdull Halim 1,2; Nazri Che Dom 1,3*; Nur M. A. S. Mat Seleei 1; Alya F. Mohd Jamili 1, Nopadol Precha 4
1, Faculty of Health Sciences, MARA Technological University (UiTM), 42300 Puncak Alam, Selangor, Malaysia
2, Setiu District Health Office, 22100 Bandar Permaisuri, Setiu, Terengganu, Malaysia
3, Integrated Mosquito Research Group (I-MeRGe), MARA Technological University (UiTM), UiTM Cawangan Selangor, 42300 Puncak Alam, Selangor, Malaysia
4, Department of Environmental Health and Technology, School of Public Health, Walailak University, Nakhon Si Thammarat, Thailand
E-mail:
nazricd@uitm.edu.my
Received: 20/09/2024
Acceptance: 16/11/2024
Available Online: 17/11/2024
Published: 01/01/2025
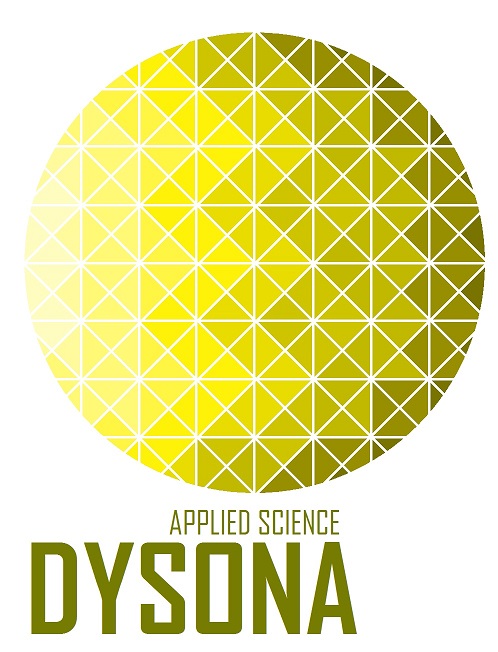
Manuscript link
http://dx.doi.org/10.30493/DAS.2024.477813
Abstract
Dengue fever, a mosquito-borne disease of growing global concern, poses significant challenges in public health, particularly in tropical and subtropical regions. The primary vectors of this disease, Aedes aegypti and Aedes albopictus, are central to the transmission of the dengue virus. With the global rise in dengue fever cases, understanding the influence of radiation application on these vectors is crucial for developing effective control strategies. Hence the present article conducted a systematic literature review on the effects of radiation on the dengue vectors. A comprehensive literature search was conducted by implementing a standardized Preferred Reporting Items for Systematic Reviews and Meta-Analyses (PRISMA). This review selected 15 relevant articles using two leading databases namely Scopus and Web of Science. Based on the thematic analysis, six themes were developed namely, 1) egg hatching response, 2) development, 3) survival, 4) longevity, 5) fecundity, and 6) behavior. The findings revealed multiple substantial contributions to practical applications and the body of knowledge. Studies have indicated that elevated radiation exposure significantly impacted the biology of dengue vectors, particularly diminishing hatching rates, survival, longevity, and fecundity, while also impairing developmental periods and insect behavior. This study highlights the potential of radiation as a tool for controlling dengue vectors. However, the varying effects of different radiation types and doses on mosquito physiology and behavior necessitate further research to optimize control strategies. This approach could contribute significantly to dengue prevention and control efforts globally.
Keywords: Aedes, Dengue vectors, PRISMA, Radiation
Introduction
Dengue fever (DF), a mosquito-borne viral disease, has emerged as a significant global public health concern [1]. The global incidence of DF cases exhibited a consistent increase, rising from 30.67 million in 1990 to 56.88 million in the past decade [2]. DF caused by the dengue virus (DENV), is primarily transmitted through the bites of infected female mosquitoes belonging to the genus Aedes, particularly Aedes aegypti and Aedes albopictus, which thrive in a wide range of ecological settings [3]. A. aegypti, often considered the principal vector, is highly adapted to urban environments and prefers to breed in artificial containers close to human dwellings. This species is known for its preference for human blood and is predominantly active during the day. On the other hand, A. albopictus, also known as the Asian tiger mosquito, is a secondary but rapidly spreading vector. It is less dependent on human habitats and has a wider range of breeding sites. Both species pose significant challenges in vector control due to their adaptability and resistance to common insecticides [4].
With the rising incidence of DF across the globe, understanding and managing these vectors have become critical in controlling the spread of the disease [5]. Recent advancements in technology and scientific research have opened new avenues for vector control, one of which is the application of radiation. Radiation, in various forms, has been studied for its impact on insects, including sterilization and changes in behavior and physiology [6]. However, the comprehensive effects of radiation on dengue vectors, specifically A. aegypti and A. albopictus, remain an area with considerable scope for research.
Complete research of the any type of radiation at all stages of Aedesmosquitoes as primary dengue vectors has not been reported. While this holometabolous insect grows and develops continuously, it goes through four different forms, each with its own morphology and degree of development [7]. The reaction to radiation may thus be expected to differ with each developmental phase, and to the degree that this is the case, the stage of development prior to exposure becomes a significant determinant. This review sought to ascertain the effects of specific doses of radiation on each stage of this species, particularly in terms of maturation and ultimate reproductive potential. Cytological effects leading to partial or total infertility and sterility were examined. A systematic literature review (SLR) was used to perform the literature review for this section. For this study, the research quality of article reviews was enhanced by implementing a standardized protocol called Preferred Reporting Items for Systematic Reviews and Meta-Analyses (PRISMA).
This SLR aims to consolidate existing knowledge on the effects of radiation application on dengue vectors. By analyzing a multitude of studies, this review seeks to provide a nuanced understanding of how different types and intensities of the applied radiation impact these vectors, including alterations in life cycle, reproductive capabilities, longevity, and behavior. The significance of this review lies in its potential to guide future research and its applicability to public health policies and vector control programs. With dengue being a leading cause of illness and death in the tropics and subtropics, effective vector control strategies are imperative. This review thus serves as a critical analysis of radiation as a viable method of controlling dengue vectors, potentially contributing to the broader efforts of dengue prevention and control.
Methodology
Literature search and study selection
The Preferred Reporting Items for Systematic Reviews and Meta-Analyses (PRISMA) was the review protocol utilized to conduct the systematic review (Fig. 1). Two databases, Scopus and Web of Science (WoS), were utilized as a source of information in this research. The main developed research question for the study was “What are the effects of radiation on the dengue vectors?”.
Systematic searching strategies
The systematic searching strategy for identifying pertinent papers in this review consists of three primary steps: identification, screening, and eligibility (Fig. 1). The initial phase involves identifying the variations of primary keywords, succeeded by the search for synonyms and related phrases utilizing dictionaries, thesauri, and encyclopedias in order to draw upon previous research and expert keywords. This approach was employed to obtain additional possibilities for the review of publications. The existing keywords and search strings were subsequently enhanced utilizing Boolean operators, phrase searching, truncation, wildcard, and field code functions within the primary databases of Scopus and Web of Science (WoS), following comprehensive access to all pertinent keywords as illustrated in Table 1.
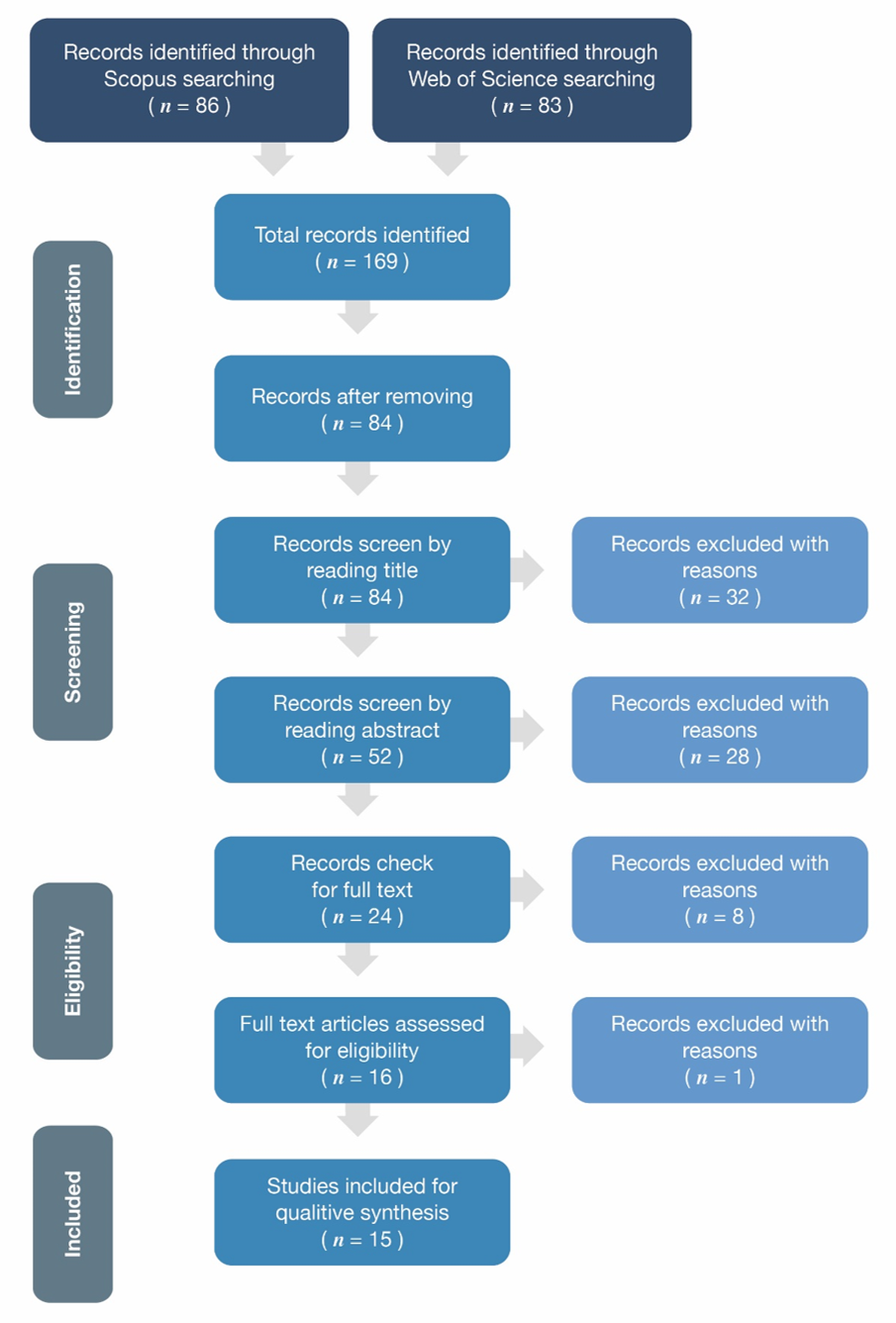
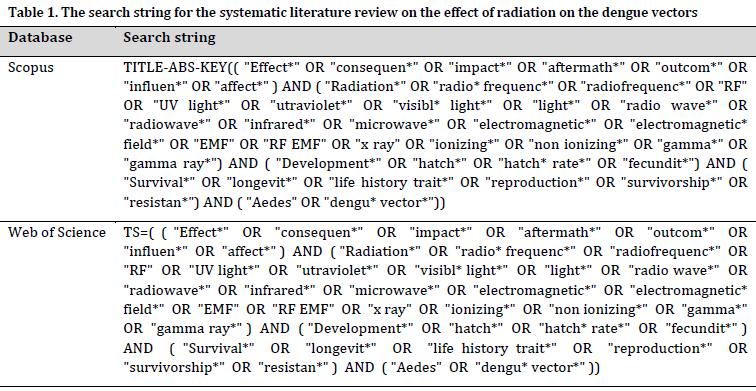
This review evaluated all the chosen articles obtained via the identification process (84 records) by using the criteria for selection, which was automatically executed using the database’s sorting function. The initial round of screening involved the elimination of duplicate items. During this process, several articles were removed, while the remaining ones underwent screening for the subsequent phase according to the inclusion and exclusion criteria established by the authors (Table 2). The initial criterion pertains to the publication type, encompassing solely articles with comprehensive empirical data published in journals as primary sources, excluding systematic reviews, meta-analyses, book chapters, and conference proceedings to ensure review quality. This review exclusively examined materials published in English to prevent any misunderstanding. A study period from 2013 to 2022 was also regarded as one of the inclusion criteria [8]. All remaining articles (52 records) were prepared for the third phase, namely eligibility. The articles were submitted to two specialists for quality evaluation to verify their content. The titles, abstracts, and primary contents of the publications were meticulously scrutinized to guarantee compliance with all inclusion criteria, thereby achieving the review’s purpose. The specialists categorized the papers into three indicators: high, moderate, and low [9]. As a result, only articles of high and moderate quality were chosen for review. To assess the quality of the articles, both experts evaluated the rank of quality according to the article’s methodology. Both authors had to reach a consensus that a minimum quality article was incorporated in the review. All arguments presented during the assessment were deliberated among them prior to determining the inclusion or exclusion criteria for subsequent analysis. Subsequently, all remaining articles chosen from the previous step (15 records) underwent qualitative analysis.
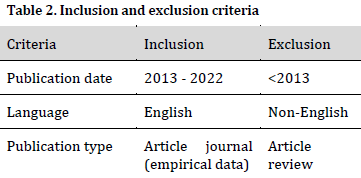
Data analysis
This review was based on an integrative analysis. This strategy necessitates the study and synthesis of many research designs, encompassing quantitative, qualitative, and mixed-method techniques considered in this review. An effective method is to analyze the integrative data utilizing qualitative or mixed-method techniques, which provide thorough comparisons across data sources [10]. The data abstraction method was executed in accordance with the study topic. The outcome data from the analyzed studies addressed the study questions and were summarized in a tabular format. The authors conducted thematic analysis to identify themes and sub-themes, thereby elucidating patterns, quantifying, grouping, and recognizing commonalities and relationships within the summarized data [11]. Thematic analysis was deemed appropriate as it effectively synthesizes integrative or mixed research designs [12]. This type of descriptive investigation diminishes flexible mode data that are integrated with other analytical techniques [13].
The preliminary phase of thematic analysis involved generating themes, during which the authors sought to discern patterns within the summarized data of all reviewed publications. All similarities identified in the reviewed data were consolidated into a single theme. Subsequently, the writers re-examined the motifs and discerned an additional sub-theme. The approach proceeds by evaluating the efficacy of the themes. In this phase, the writers meticulously examined all primary and secondary themes to guarantee the efficacy and precision of data representation. Throughout this procedure, certain subjects may be omitted based on the inclusion criteria established by the authors. The subsequent phase involved designating appropriate titles for the primary themes and their corresponding sub-themes. During the theme generation process, this technique engaged authors to ascertain the most pertinent themes aligned with the purpose of this review. The authors deliberated on ideas, inconsistencies, similarities, and associations about data interpretation until consensus on theme development was achieved. Subsequently, the formulated themes and their sub-themes were presented to two expert panelists, both of whom specialize in qualitative research. All experts were requested to categorize the automatically created topics and determine the relevance and appropriateness of the themes and sub-themes for the review outcomes.
Results
General findings of the review
Overall, 15 articles were selected for inclusion in the systematic review (Table 3).
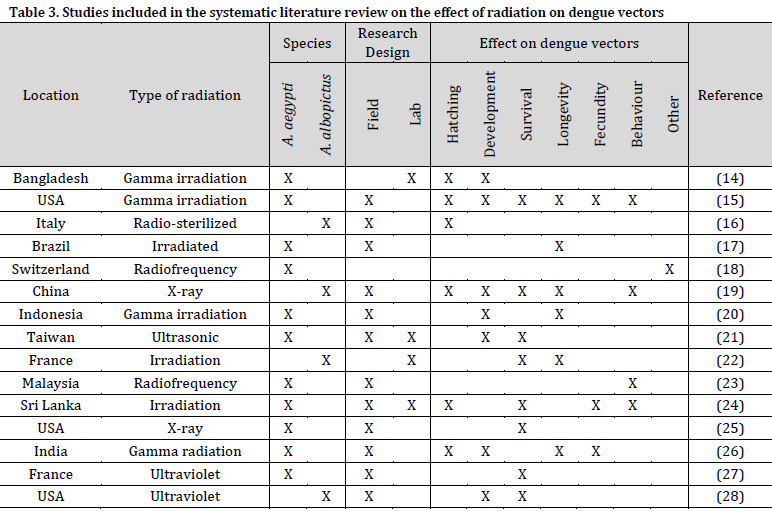
Amongst the selected articles, two studies were conducted in USA [15][25][28], two in France [23][27], and one in each of Bangladesh [14], Italy [16], Brazil [17], Switzerland [18], China [19], Indonesia [20], Taiwan [21], Malaysia [23], Sri Lanka [24], and India [26]. Eleven articles were conducted with A. Aegypti being the main subject, while the four other manuscripts studied A. albopictus. Moreover, eleven studies were conducted under field conditions, highlighting the importance of the collective knowledge provided to investigate the potential of radiation application on dengue vectors. Six themes were created as a result of the thematic analysis: hatching response, development, survival, longevity, fecundity, and behavior.
Egg hatching response
Electromagnetic radiation can influence the hatching rate of Aedes mosquito eggs. The urgency of this issue has escalated, as a successful hatching phase will lead to an increase in vector density [29]. The reports indicate that the hatching rate of A. aegypti decreased significantly by up to 55% following exposure to gamma irradiation at a dose of 10 Gy [14]. Additionally, male eggs of A. aegypti irradiated with doses between 3 and 50 Gy exhibited significantly reduced hatchability compared to controls, while doses of 1 and 2 Gy showed no effect [26]. Another study showed that egg hatch rates of A. aegypti decreased at doses exceeding 50 Gy in comparison to doses below 40 Gy, which may provide support for this observation [24]. A significant difference in hatchability was observed, with the control group achieving 96.80% and the irradiated eggs of the F1 generation attaining only 93.01% following the application of 50 Gy radiation [24][26]. No correlations were identified between male pupal and adult irradiation and the female egg count of A. albopictus [19]. However, an increase in radiation exposure was associated with a significant decrease in hatching rates. A. aegypti egg hatching was observed in several irradiation treatments (0-34 Gy), with one duplicate cage containing viable eggs at high doses during the initial gonotrophic cycle [15]. Nevertheless, none of the females exposed to radiation produced viable eggs during the second gonotrophic cycle. Females exposed to 20 Gy as pupae exhibited lower egg hatching rates compared to non-irradiated females. In the 30-Gy treatment group, one egg laid by a duplicate cage during the second gonotrophic cycle did not hatch [15].
Mosquito development
A reduced developmental period leads to an increased availability of vectors, and therefore, poses a significant threat to the human population. In this context, numerous studies have demonstrated that radiation, including gamma radiation, has significantly hindered the formation of the dengue vector. A gradual decline in the adult appearance of A. aegypti across all three generations, beginning at 3 Gy of irradiation, accompanied by a minor increase in later generations relative to the first generation (F1) was identified [26]. The emergence rate decreased from 97.50% at 0 Gy (control) to 65.83% at 100 Gy [20]. The same report showed that the emergence rate of A. aegypti decreased with higher gamma irradiation doses. Nevertheless, the difference between the control and the 70 Gy dose was not significant, whereas substantial differences were observed at the 80 and 100 Gy doses compared to the control [20]. The proportion of A. aegypti adults that successfully emerged from pupae was not significantly influenced by the dose of gamma irradiation administered to the pupae [15]. The emergence rates of pupal A. albopictus across all age groups and X-ray radiation dosages were not significantly different from the controls [19]. Moreover, UV-B radiation conditions had no significant influence on the development periods of A. albopictus; however, there was a minor impact on metabolic rate [28]. As for ultrasonic radiation, the tested sound waves resonance resulted in the rupture of A. aegypti larvae. Consequently, 98% of the larvae and pupae perished, while the remaining 2% survived but exhibited morphological deformities, leading to imminent death [21].
Survival
In this context, some reports showed no significant difference in the percentage of pupal survival to adulthood for male and female A. aegypti subjected to irradiation doses between 0 and 40 Gy [24]. However, this study showed that 80 Gy dose markedly diminished male survival (81.11%) compared to control (96.22%). Similarly, A. albopictus males subjected to 40 to 70 Gy of radiation had survival rates comparable to those not exposed [22].
A separate study indicated that the high dosage of ethanol exhibited a significant detrimental impact of x-ray radiation on the survival of A. aegypti mosquitoes, whereas those treated with lesser amounts showed no effect from x-ray radiation [25]. Females of A. aegypti subjected to gamma irradiation dosages over 10 Gy had considerably reduced lifespans compared to nonirradiated controls, with the most pronounced mortality observed between 40 and 50 Gy [15]. It was reported that the death rate of A. aegypti larvae increased with prolonged ultrasonic exposure duration and a constant distance from the transducer [21]. After 30 seconds of exposure, the ultrasonic wave spectrum at a frequency of 18 to 30 kHz resulted in 100% mortality of A. aegypti larvae and pupae at a distance of 20 cm from the transducer, and 100% mortality of instars L1, L2, and L3 at a distance of 35 cm. At a distance of 60 cm, it requires 180 seconds to eliminate all pupae. A study by [22] indicated that the mortality rates of male A. albopictus were significantly elevated compared to females, irrespective of irradiation status. However, an immediate provision of sugar could markedly reduce mortality rates. Furthermore, the survival of A. albopictus has been shown to be influenced by exposure to ultraviolet light in comparison to non-ultraviolet conditions [28].
Longevity
According to [20], gamma irradiation exposure significantly decreased A. aegypti longevity. However, exposure to 4 Gy of gamma radiation increased the life expectancy of parental (F0) males and first generations (F1) males by 10.56 days (27.38%) and 8.66 days (22.92%), respectively, when compared to controls. Another report stated that F0 of A. aegypti suffer a decline in longevity starting from 6 Gy of gamma radiation [26]. For instance, the greatest drop in longevity (19.61 days, 69.9%) was observed in F0 at a radiation dose of 50 Gy. Based on the findings of a study conducted by [19], A. albopictus mosquito’s lifespan decreased with the increased x-ray doses. When compared to controls, irradiation at 40 Gy and 60 Gy significantly reduced male and female longevity in pupae and adult phases. Adult males lived an average of 22 days after 40 Gy exposure and 13 days after 60 Gy exposure. Under the same treatments, females lived an average of 29 days and 19 days, respectively. Furthermore, according to another investigation on irradiation exposure, when sugar supply was delayed in sterile male treatments, independent of the presence of A. albopictus females, the mean lifetime was significantly lowered. However, when sterile males were provided sugar upon emergence and no mating activity was allowed, their mean longevity was comparable to that of wild males (15.5 ±1.1 days) [22].
Fecundity
Evaluating the induced effects of persistent radiation with varied dosages across consecutive generations is thought necessary in order to gain a clear perspective of the repercussions of exposing a population to radiation. Little is known about the influence of radiation on adult female A. aegypti development and behavior since, historically, males have received more attention than females. According to [26], fertility of A. aegypti mosquito was unaffected by dosages ranging from 1 to 25 Gy. The fertility of the F0 generations, however, was only impacted by larger doses, such as 30, 35, 40, 45, and 50 Gy while F1, F2, and F3 generations were unaffected. In another study, all the gamma irradiation dosages reduced female A. aegypti fecundity by using a dosage up to 110 Gy [15]. The results showed that egg laying had completely stopped at radiation doses > 20 Gy. Additionally, radiation dosages of more than 20 Gy dramatically decreased the female fecundity [15]. On the other hand, [24] reported a reduction in fertility from 87.66% to 0% in 80 Gy irradiated male of A. aegypti mated with non-irradiated females. Similarly, female fertility decreased from 92.53% to 0 in treatments involving 70-80 Gy irradiated females when mated with non-irradiated males.
Behavior
Various studies reported the impact of radiation application on dengue vectors behavior. Flying ability notably decreased in irradiated A. aegypti males and females compared to the non-irradiated controls [24]. On the other hand, no significant differences in terms of escape rates were noted between non-irradiated and irradiated mosquitos. In another study, all irradiated mosquito groups showed lower adult escape rates for males and females than non-irradiated mosquitoes [23]. Investigating mating behavior also showed that mating competitiveness (C index) significantly decreased as gamma irradiation dose rose in all developmental stages/groups, including the pupal stage of A. aegypti [20]. Similarly, another study reported that x-ray radiation during A. albopictus pupal and adult phases reduced competitiveness index C in comparison to controls. As a consequence, 1.8–1.9 radiated males were needed to cause the same sterility [19]. As for blood feeding behavior, it was found that a radiation dose ≥30 Gy can significantly decrease blood feeding events [15].
Discussion
Various studies showed that Aedes mosquito egg hatchability had significantly decreased when exposed to various radiation. Similar observations were reported in Anopheles pharoensis and Anopheles arabiensis as radiation caused a considerable reduction in hatchability [30]. Radiation, especially ionizing radiation, can harm a cell’s genetic makeup resulting in DNA mutations or structural damage especially chromosomal damage in mosquito eggs. These changes negatively impact the normal developmental processes and can, ultimately, result in the death of an embryo [31].
Developmental delays can result from radiation exposure in Aedes mosquitoes. Compared to non-irradiated individuals, irradiated larvae or pupae could develop more slowly. The timing of the emergence of adults can be impacted by this development in terms of physical anomalies, defects in organ development, or physical deformities, which may also disrupt population dynamics. For example, higher metabolic rates for mosquito larvae exposed to high levels of UV radiation, particularly with increasing exposure time, are likely to signify greater energy expenditure to carry out fundamental biological activities, including development and immune functioning. It is likely that, similar to other aquatic and semi-aquatic life forms, mosquito larvae need to use more energy under full-sun circumstances [32]. As a comparative example with other species of mosquitoes, a dramatic increase in the metabolic rate of Culex tarsalis larvae was observed after the emergence in outdoor setting and being exposed to UV radiation [33]. Histological examinations revealed that ultra-violet radiation can cause partial paralysis, abdominal segment disintegration, and cuticle damage in mosquito larvae [34] and inflect DNA, proteins, and membranes damages [35][36]. Since hormones play a significant role in the length of insect development and insect body size [37], radiation, as any environmental stressor, can influence the hormonal balance. For instance, the prothoracicotropic hormone is crucial for the release of ecdysone, a steroid hormone that influences the size and length of larval development in a variety of insects, including mosquitoes [38]. By affecting prothoracicotropic hormone and ecdysteroid metabolism, radiation has also been demonstrated to retard Tribolium castaneum’s metamorphosis [39].
High radiation exposure may result in an increase in mosquito mortality. A strong radiation dose can cause a significant damage at the cellular, tissue, and organ level. In particular, if the radiation exposure is high, this may lead to decreased general fitness and increased mortality risk. From a molecular perspective, ultrasounds radiation at the cellular level can result in membrane blubbing, cell shrinkage, nucleus fragmentation, chromatin condensation, and the fragmentation of apoptotic bodies [40]. Unsurprisingly, the morphological examination of treated and deceased A. aegypti larvae supported the theory that the acoustic energy from the transducer ultrasound ruptured the mosquito tracheal trunk in this species [21]. It should also be emphasized that, regardless of the source or visibility, mosquitoes can detect ultrasound at a wavelength range of 38-44 kHz [41]. Environmental pressures are frequently displayed and identified in insects by the release of hormones such as cortisol, epinephrine, and octopamine [42]. Stress hormone release as a result of radiation has also been linked to decreased survival in red flour beetle (Tribolium castaneum) [39].
Radiation-induced male sterility is among the most promising strategy in controlling Aedes mosquitos in natural settings. However, the radiation damage to somatic cells lowers male mate competition and longevity [16]. Therefore, in order to increase the effectiveness of radiation control, an increase in the numbers of released sterile males should be taken into consideration to compensate for the decreased mating competitiveness observed in these males. Similarities are observed in other insects, as the male Mediterranean fruit fly (Ceratitis capitata) in Guatemala became less competitive in mating, but the wild population was successfully controlled by increasing the release ratio of sterile males to 100:1 [43]. As the dose exceeds 80 Gy, some anopheline species, including Anopheles stephensi, Anopheles pharoensis, and Anopheles gambiae, have shown irradiation-induced reductions in longevity [44].
The lack of fecundity is primarily defined as the failure to lay eggs, inability of male to generate functional sperm, and incapacity to mate [45]. Significant levels of radiation can reduce mosquito Aedes fecundity. A study on Anopheles pharoensis revealed a considerable increase in fecundity at 10 Gy, followed by no effects at dosages between 15 and 20 Gy, and then a significant drop in fecundity was seen at 70 Gy [46]. Radiation can damage reproductive organs, interfere with the development of gametes (eggs and sperm), or hinder the normal fusion of gametes during fertilization. Additionally, somatic cells are harmed by irradiation, which lowers male mate competition and longevity [16]. Female gonads of A. aegypti are more vulnerable to radiation than male gonads, and female adults were completely infertile when irradiated at levels of 35 Gy as pupae [47].
The impact of radiation on Aedes mosquito behavior varies according to the dosage, exposure duration, and species-specific characteristics. Radiation exposure to biological materials can damage insect cells, perhaps adversely affecting adult performance [44]. In addition to the direct tissue damage inflected by radiation, it is evident that it may impair female host-seeking and blood feeding behavior [48]. Moreover, radiation has been shown to influence the midguts of other insect species [49], and it is plausible that midgut damage could disrupt the feeding motivation of females. Regarding flight capability and resting behavior, previous studies on Anopheles gambiae mosquitoes behavior suggest that the resting behavior observed during most of the experiment is mostly induced by ambient carbon dioxide (CO2) [50]. The intermittent appearance in the spectrum is likely attributable to the pervasive CO2 in the laboratory environment. This experiment indicates that the behavior of the insects within the chamber is inconsistent. Numerous insect species, such as fruit flies, honey bees, and cockroaches, have been documented to be affected by frequencies emitted by telecommunication towers within the range of 800 to 2600 MHz [51]. Conversely, irradiation did not affect the flying capability of male and female A. aegypti mosquitoes, which may be attributed to the inadequate sensitivity of the flight apparatus [24].
Conclusion
This systematic literature review has critically evaluated the existing research (between 2013 and 2022) on the impact of radiation on A. aegypti and A. albopictus, the primary vectors of dengue fever. The analysis reveals that radiation, particularly gamma rays, X-rays, and ultrasonic waves, can significantly influence the life cycle, survival, behavior, and reproductive capabilities of these mosquitoes. Key findings demonstrate that lower doses of gamma irradiation can reduce egg hatchability and longevity in Aedes aegypti, while higher doses notably impact adult emergence, survival, and fecundity. This suggests that radiation could be an effective tool in reducing vector populations and potentially interrupting the transmission cycle of dengue fever. Furthermore, the observed alterations in mosquito behavior, such as reduced flight ability and mating competitiveness at higher radiation doses, underline the potential of radiation in vector control strategies. However, the review also highlights the complexity of radiation effects, which vary with the type and dosage of radiation, and the developmental stage of the mosquito. This underscores the need for further research to optimize radiation parameters for effective vector control without causing undue environmental impact. Limitations of this review include the variability in experimental designs and radiation parameters across studies, which may affect the generalizability of the findings. In conclusion, while radiation presents a promising avenue for dengue vector control, a careful and considered approach is necessary to fully harness its potential. Continued research is essential to refine radiation-based strategies, ensuring their safety, effectiveness, and sustainability in the global fight against dengue fever.
Acknowledgement
The authors would like to convey their heartfelt appreciation to all organizations engaged in this effort. We also would like to offer thanks to the Research Management Centre, Universiti Teknologi MARA (UiTM) for the technical assistance rendered.
References
- Rozilawati H, Masri M, Tanaselvi K, TH M, Zairi J, Nazni W, Lee H. Life table characteristics of Malaysian strain Aedes albopictus (Skuse). Serangga. 2018;22:85-121.
- Bhatt S, Gething PW, Brady OJ, Messina JP, Farlow AW, Moyes CL, Drake JM, Brownstein JS, Hoen AG, Sankoh O, Myers MF. The global distribution and burden of dengue. Nature. 2013;496(7446):504-7. DOI
- Rao MR, Padhy RN, Das MK. Episodes of the epidemiological factors correlated with prevailing viral infections with dengue virus and molecular characterization of serotype-specific dengue virus circulation in eastern India. Infect. Genet. Evol. 2018;58:40-9. DOI
- Ferede G, Tiruneh M, Abate E, Kassa WJ, Wondimeneh Y, Damtie D, Tessema B. Distribution and larval breeding habitats of Aedes mosquito species in residential areas of northwest Ethiopia. Epidemiol. Health. 2018;40. DOI
- Reyes-Castro PA, Castro-Luque L, Diaz-Caravantes R, Walker KR, Hayden MH, Ernst KC. Outdoor spatial spraying against dengue: A false sense of security among inhabitants of Hermosillo, Mexico. PLoS Negl. Trop. Dis. 2017;11(5): e0005611. DOI
- Balmori A. Electromagnetic radiation as an emerging driver factor for the decline of insects. Sci. Total Environ. 2021;767:144913. DOI
- Foster WA, Walker ED. mosquitoes (Culicidae). Medical and Veterinary Entomology 3rd ed. Academic press. 2019, 261-325. DOI
- Okoli C. A guide to conducting a standalone systematic literature review. Commun. Assoc. Inf. Syst. 2015;37. DOI
- Petticrew M, Roberts H. Systematic reviews in the social sciences: A practical guide. John Wiley & Sons. 2008. DOI
- Whittemore R, Knafl K. The integrative review: updated methodology. J. Adv. Nurs. 2005;52(5):546-53. DOI
- Braun V, Clarke V. Using thematic analysis in psychology. Qual. Res. Psychol. 2006;3(2):77. DOI
- Flemming K, Booth A, Garside R, Tunçalp Ö, Noyes J. Qualitative evidence synthesis for complex interventions and guideline development: clarification of the purpose, designs and relevant methods. BMJ Glob. Health. 2019;4(1) :e000882. DOI
- Vaismoradi M, Turunen H, Bondas T. Content analysis and thematic analysis: Implications for conducting a qualitative descriptive study. Nurs. Health Sci. 2013;15(3):398-405. DOI
- Akter H, Khan SA. Sensitivity of immature stages of dengue causing mosquito, Aedes aegypti (L.) to gamma radiation. J. Entomol. 2014;11(2):56-67. DOI
- Aldridge RL, Kline J, Coburn JM, Britch SC, Boardman L, Hahn DA, Chen C, Linthicum KJ. Gamma-irradiation reduces survivorship, feeding behavior, and oviposition of female Aedes aegypti. J. Am. Mosq. Control Assoc. 2020;36(3):152-60. DOI
- Bellini R, Medici A, Puggioli A, Balestrino F, Carrieri M. Pilot field trials with Aedes albopictus irradiated sterile males in Italian urban areas. J. Med. Entomol. 2013;50(2):317-25. DOI
- Culbert NJ, Maiga H, Somda NS, Gilles JR, Bouyer J, Mamai W. Longevity of mass-reared, irradiated and packed male Anopheles arabiensis and Aedes aegypti under simulated environmental field conditions. Parasites Vectors. 2018;11(1):1-8. DOI
- De Borre E, Joseph W, Aminzadeh R, Müller P, Boone MN, Josipovic I, Hashemizadeh S, Kuster N, Kühn S, Thielens A. Radio-frequency exposure of the yellow fever mosquito (A. aegypti) from 2 to 240 GHz. PLoS Comput. Biol. 2021;17(10): e1009460. DOI
- Du W, Hu C, Yu C, Tong J, Qiu J, Zhang S, Liu Y. Comparison between pupal and adult X-ray radiation, designed for the sterile insect technique for Aedes albopictus control. Acta Trop. 2019;199:105110. DOI
- Ernawan B, Anggraeni T, Yusmalinar S, Ahmad I. Investigation of developmental stage/age, gamma irradiation dose, and temperature in sterilization of male Aedes aegypti (Diptera: Culicidae) in a sterile insect technique program. J. Med. Entomol. 2022;59(1):320-7. DOI
- Kalimuthu K, Tseng LC, Murugan K, Panneerselvam C, Aziz AT, Benelli G, Hwang JS. Ultrasonic technology applied against mosquito larvae. Appl Sci. 2020;10(10):3546. DOI
- Oliva CF, Maier MJ, Gilles J, Jacquet M, Lemperiere G, Quilici S, Vreysen MJ, Schooneman F, Chadee DD, Boyer S. Effects of irradiation, presence of females, and sugar supply on the longevity of sterile males Aedes albopictus (Skuse) under semi-field conditions on Reunion Island. Acta Trop. 2013;125(3):287-93. DOI
- Poh AH, Moghavvemi M, Shafiei MM, Leong CS, Lau YL, Mahamd Adikan FR, Bakhtiari M, Abdulla Hassan MA. Effects of low-powered RF sweep between 0.01-20 GHz on female Aedes aegypti mosquitoes: A collective behaviour analysis. PloS One. 2017;12(6): e0178766. DOI
- Ranathunge T, Harishchandra J, Maiga H, Bouyer J, Gunawardena YN, Hapugoda M. Development of the Sterile Insect Technique to control the dengue vector Aedes aegypti (Linnaeus) in Sri Lanka. PLoS One. 2022;17(4): e0265244. DOI
- Rodriguez SD, Brar RK, Drake LL, Drumm HE, Price DP, Hammond JI, Urquidi J, Hansen IA. The effect of the radio-protective agents ethanol, trimethylglycine, and beer on survival of X-ray-sterilized male Aedes aegypti. Parasites Vectors. 2013;6:1-8. DOI
- Shetty V, Shetty NJ, Harini BP, Ananthanarayana SR, Jha SK, Chaubey RC. Effect of gamma radiation on life history traits of Aedes aegypti (L.). Parasite Epidemiol. Control. 2016;1(2):26-35. DOI
- Tetreau G, Chandor-Proust A, Faucon F, Stalinski R, Akhouayri I, Prud’homme SM, Raveton M, Reynaud S. Contrasting patterns of tolerance between chemical and biological insecticides in mosquitoes exposed to UV-A. Aquat. Toxicol. 2013;140:389-97. DOI
- Villena OC, Momen B, Sullivan J, Leisnham PT. Effects of ultraviolet radiation on metabolic rate and fitness of Aedes albopictus and Culex pipiens mosquitoes. PeerJ. 2018;6: e6133. DOI
- Patz JA, Campbell-Lendrum D, Holloway T, Foley JA. Impact of regional climate change on human health. Nature. 2005;438(7066):310-7. DOI
- Helinski ME, Parker AG, Knols BG. Radiation-induced sterility for pupal and adult stages of the malaria mosquito Anopheles arabiensis. Malar. J. 2006;5:1-10. DOI
- LaChance LE. The induction of dominant lethal mutations in insects by ionizing radiation and chemicals-as related to the sterile male technique of insect control. Genet. Insect Vectors Dis. 1967;21:617-50.
- Häder DP, Kumar HD, Smith RC, Worrest R. Effects of solar UV radiation on aquatic ecosystems and interactions with climate change. Photochem. Photobiol. Sci. 2007;6(3):267-85. DOI
- Gray EM, Bradley TJ. Metabolic rate in female Culex tarsalis (Diptera: Culicidae): age, size, activity, and feeding effects. J. Med. Entomol. 2003 1;40(6):903-11. DOI
- MacGregor ME. Certain pathological effects of ultra-violet radiation on mosquito larvae and pupae. Proc. R. Soc. Lond. Ser. B Biol. Sci. 1932;112(774):27-38. DOI
- Wu H, Gao K, Wu H. Responses of a marine red tide alga Skeletonema costatum (Bacillariophyceae) to long-term UV radiation exposures. J. Photochem. Photobiol. B Biol. 2009;94(2):82-6. DOI
- Jayachandran G, Fallon AM. The mosquito ribonucleotide reductase R2 gene: ultraviolet light induces expression of a novel R2 variant with an internal amino acid deletion. Insect Mol. Biol. 2004;13(3):231-9. DOI
- McBrayer Z, Ono H, Shimell M, Parvy JP, Beckstead RB, Warren JT, Thummel CS, Dauphin-Villemant C, Gilbert LI, O’Connor MB. Prothoracicotropic hormone regulates developmental timing and body size in Drosophila. Dev. Cell. 2007;13(6):857-71. DOI
- Zhang Q, Denlinger DL. Molecular structure of the prothoracicotropic hormone gene in the northern house mosquito, Culex pipiens, and its expression analysis in association with diapause and blood feeding. Insect Mol. Biol. 2011;20(2):201-13. DOI
- Sang W, Yu L, He L, Ma WH, Zhu ZH, Zhu F, Wang XP, Lei CL. UVB radiation delays Tribolium castaneum metamorphosis by influencing ecdysteroid metabolism. PLoS One. 2016;11(3):e0151831. DOI
- Tian ZM, Wan MX, Lu MZ, Wang XD, Wang L. The alteration of protein profile of Walker 256 carinosarcoma cells during the apoptotic process induced by ultrasound. Ultrasound Med. Biol. 2005;31(1):121-8. DOI
- Mohankumar D. Ultrasound and insects. Electronics and Animal Science. 2010.
- Farooqui T. Review of octopamine in insect nervous systems. Open Access Insect Physiol. 2012;4. DOI
- Rendón P, McInnis D, Lance D, Stewart J. Medfly (Diptera: Tephritidae) genetic sexing: large-scale field comparison of males-only and bisexual sterile fly releases in Guatemala. J. Econ. Entomol. 2004;97(5):1547-53. DOI
- Sharma VP, Razdan RK, Ansari MA. Anopheles stephensi: effect of gamma-radiation and chemosterilants on the fertility and fitness of males for sterile male releases. J. Econ. Entomol. 1978;71(3):449-52. DOI
- Lance DR, McInnis DO. Biological basis of the sterile insect technique. Sterile Insect Technique. 2021:113-42.
- Abdel-Malek AA, Tantawy AO, Wakid AM. Studies on the eradication of Anopheles pharoensis Theobald by the sterile-male technique using Cobalt-60. I. Biological effects of gamma radiation on the different developmental stages. J. Econ. Entomol. 1966;59(3):672-8. DOI
- Asman SM, Rai KS. Developmental effects of ionizing radiation in Aedes aegypti. J. Med. Entomol. 1972;9(5):468-78. DOI
- Ali, SR., Rozeboom, LE. Observations on sterilization of Anopheles (C.) albimanus Wiedemann by x-irradiation. Mosq. News. 1972, 32(4), 574-579.
- Dyck VA, Hendrichs J, Robinson AS. Sterile insect technique: principles and practice in area-wide integrated pest management. Taylor & Francis. 2021.
- Webster B, Lacey ES, Cardé RT. Waiting with bated breath: opportunistic orientation to human odor in the malaria mosquito, Anopheles gambiae, is modulated by minute changes in carbon dioxide concentration. J. Chem. Ecol. 2015;41:59-66. DOI
- Lázaro A, Chroni A, Tscheulin T, Devalez J, Matsoukas C, Petanidou T. Electromagnetic radiation of mobile telecommunication antennas affects the abundance and composition of wild pollinators. J. Insect Conserv. 2016;20:315-24. DOI
Cite this article:
Nik Abdull Halim, N. M. H., Che Dom, N., Mat Seleei, N. M. A. S., Mohd Jamili, A. F., Precha, N. Radiation effects on dengue vectors: A systematic review. DYSONA – Applied Science, 2025;6(1): 160-171. doi: 10.30493/das.2024.477813