Jamiu F. Atanda 1*; Lateef E. Yahaya 1; Mikhail O. Nafiu 2; Mustapha Kazeem 1; Abiodun O. Ajewole 1
1, Department of Value Addition Research, Cocoa Research Institute of Nigeria, Ibadan, Nigeria
2, Department of Biochemistry, Faculty of Life Sciences, University of Ilorin, Ilorin, Nigeria
E-mail:
jamiuatandaonly@yahoo.com
Received: 26/10/2023
Acceptance: 20/11/2023
Available Online: 21/11/2023
Published: 01/04/2024
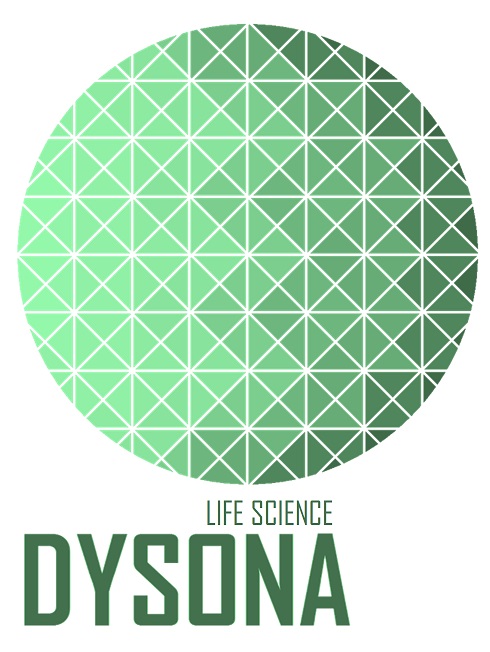
Manuscript link
http://dx.doi.org/10.30493/DLS.2023.422394
Abstract
The aim of this study was to assess the potential adverse effects of tea seed oil (TSO) on specific indicators of renal function and antioxidant levels in male albino rats. The administration of TSO was conducted orally, with varying daily doses (0.15, 0.3, 0.45, and 0.6 ml kg-1 body weight) to four distinct groups of rats over a period of 28 days. In contrast, a control group received a daily dosage of 0.1 ml of distilled water. A thorough examination was conducted on a range of parameters, encompassing body weight, relative kidney weight, electrolyte levels, kidney function markers, and indicators of antioxidant status. No signs of toxicity or mortality were observed during the entire duration of the study. Increased doses of TSO exhibited favorable impacts on body weight. Nevertheless, the administration of TSO did not exhibit any correlation with alterations in the relative weight of the kidneys in the rats. Despite the significant increase in serum concentrations of K+ and HCO3– ions, the administration of higher TSO doses did not result in electrolyte imbalances in the rats, as all measured electrolytes remained within the normal ranges. There were no statistically significant differences observed in the levels of urea and creatinine between the groups that received TSO and the control group. The administration of TSO resulted in a notable reduction in malondialdehyde (MDA) concentrations, as well as a dose-dependent elevation in the activities of antioxidant enzymes such as superoxide dismutase (SOD) and glutathione peroxidase (GPx), which was accompanied by an increase in glutathione (GSH) levels. The findings of this study indicate that the consumption of TSO may have the potential to provide health benefits by improving kidney function and reducing oxidative stress.
Keywords: Tea seed oil, Camellia sinensis, Electrolytes, Oxidative stress, Renal function
Introduction
Throughout generations, humans have extensively investigated numerous medicinal plants for their potential therapeutic properties, even in the absence of scientific evidence to support their efficacy in treating or preventing various ailments. Contemporary medical science, through an in-depth understanding of the therapeutic capacities inherent in diverse botanical species, has elucidated that specific bioactive constituents present within these plants, including saponins, tannins, alkaloids, flavonoids, and terpenoids, are accountable for the observed remedial attributes [1]. Nowadays, the demand for and acceptance of medicinal plants in disease treatment is experiencing a notable increase [2]. This claim can be ascribed to the convenient accessibility, affordability, minimal adverse effects of medicinal plants, and their extensive range of botanical constituents that can be utilized for the production of numerous therapeutic medications [3].
Tea (Camellia sinensis) is not only consumed worldwide as a beverage known for its astringent taste and pleasant aroma but it is also utilized for its therapeutic attributes [4]. The tea seed, typically regarded as a byproduct of the tea plant, possesses a significant abundance of oil. Tea seed oils (TSOs) are composed of unsaturated fatty acids, including oleic and linoleic acids [5]. TSOs are edible and their health-promoting potentials have been substantiated through various scientific experiments as documented in several publications.
Several plant species that are commonly consumed as food or used for medicinal purposes have been found to contain toxic attributes. These toxic properties can be attributed to the chemical constituents present in these plants. These plants have the potential to present various health risks, including severe organ damage or even fatality, to individuals who consume them. An illustration can be seen in the case of cassava (Manihot esculenta), a widely consumed crop in Africa that serves as a primary source of dietary carbohydrates. It is worth noting that cassava contains cyanogenic glycoside, a compound that has been linked to numerous incidents of foodborne illnesses [6]. Ginkgo (Ginkgo biloba), a plant commonly employed for its anti-inflammatory properties in the treatment of bronchoconstriction during asthmatic episodes and its potential to enhance cognitive function in individuals with dementia, has been associated with adverse effects including intracranial hemorrhages, bilateral subdural hematomas, and bleeding [7]. Given the aforementioned factors, it is crucial to ascertain the toxicity of TSO within a biological context. The present study aims to examine the potential toxicological impacts of TSO on the renal electrolyte balance and antioxidant status in male rats. The findings derived from this study would provide objective data or knowledge regarding the safety of TSO consumption to prospective consumers.
Materials and Methods
Tea seed oil extraction
Tea (Camelia sinensis) seeds were obtained from the Mambilla substation of the Cocoa Research Institute of Nigeria (CRIN), Kusuku, Taraba State, Nigeria. The tea seeds underwent a process of air-drying, de-husking, and pulverization into a fine powder using an electric blender (Model QBL-20L330, Qlink, Taiwan). A 50 g sample of pulverized tea seeds was subjected to extraction using a Soxhlet apparatus, employing 400 milliliters of petroleum ether as the extracting solvent for a duration of 8 hours. Following the extraction process, the solvent was removed through rotary evaporation conducted at a temperature of 60 °C, utilizing a nitrogen stream.
Animals and experimental design
A total of thirty-five (35) adult male albino rats, with weights ranging from 150-200g, were acquired from the animal facility at the College of Medicine, University of Lagos, Lagos, Nigeria. The animals were placed in adequately ventilated plastic enclosures and given a period of 14 days to acclimate prior to the initiation of the experiment. During this time frame, the rats were provided with commercially available rat feed and unrestricted access to water.
The animals were allocated in a random manner to five groups (A-E), with each group consisting of seven rats. The rats in group A, which served as the control group, were administered 0.1 ml kg-1 b.wt of distilled water orally. Rats in experimental groups B, C, D, and E were subjected to varying doses of TSO, specifically 0.15, 0.3, 0.45, and 0.6 ml kg-1 b.wt, respectively. These doses were administered once daily over a period of 28 days. The body weights of the rats were measured prior to the initiation of administration and at the time of sacrifice in order to assess any variations in weight. The animals were also monitored during the duration of the experiment for clinical indications of toxicity, including alterations in behavior, physical appearance, or any negative effects.
Blood and kidney tissue samples collection
Upon completion of the experiment, the rats underwent an overnight fasting period and were subsequently euthanized through cervical dislocation. Blood samples were obtained from each rat via cardiac puncture and collected into sterile plain tubes. The blood samples were permitted to coagulate, subjected to centrifugation at a speed of 4000 rpm for a duration of 10 minutes, and the resulting sera were isolated into sterile sample containers for subsequent analysis.
The kidney of each rat was surgically removed, rinsed with saline solution at a low temperature, and weighed to determine the relative weight of the organ. The formula provided below was utilized to calculate the relative organ weight (ROW) for each individual rat:
ROW (%) = Absolute organ weight (g) / Total body weight at sacrifice (g) x 100
Determination of serum electrolytes
The concentrations of sodium, potassium, chloride, and bicarbonate ions in the collected serum samples were measured [8]. The determination of creatinine was conducted using a modified Jaffe’s method [9]. On the other hand, the determination of urea content was carried out utilizing the urease/Berthelot method [10].
Determination of antioxidant activity
The kidneys were promptly minced and homogenized in a solution of ice-cooled 50 mM Tris-HCl with 2 mM EDTA (pH 7.4) to produce homogenates with a concentration of 10% (w/v). The homogenates underwent centrifugation at 16,000 g and 4°C for 10 minutes. The resulting supernatant was utilized for the assessment of antioxidant properties.
The determination of catalase (CAT) activity was conducted using the technique described in [11]. The estimation of superoxide dismutase (SOD) activity was conducted using the pyrogallol autoxidation method as described by [12]. The method for determining reduced glutathione (GSH) involved the utilization of the 5,5′-dithiobis(2-nitrobenzoic acid) (DTNB) recycling technique, as outlined in [13]. The determination of glutathione peroxidase (GPx) was conducted using the method described by [14]. The concentration of malondialdehyde (MDA) was determined using the experimental procedure outlined in [15].
Statistical analysis
The data were reported in the format of Mean ± Standard Deviation, utilizing the statistical software SPSS version 27. Statistical analysis was conducted to ascertain notable disparities in the means of the control and experimental groups. This involved employing a one-way analysis of variance (ANOVA) followed by a post-hoc Tukey test at P<0.05.
Results
The effect of tea seed oil on total body weight and relative kidney weight
There was no statistically significant difference observed in total body weight between group B or C (0.15 and 0.3 ml kg-1 b.wt TSO, respectively) and the control group (A). However, significant differences were observed in the total body weight of rats in groups D and E (0.45 and 0.6 ml kg-1 b.wt TSO, respectively) compared to the control group (Fig. 1). On the other hand, there were no notable distinctions observed among the groups in relation to the relative weight of the kidneys (Fig. 2).
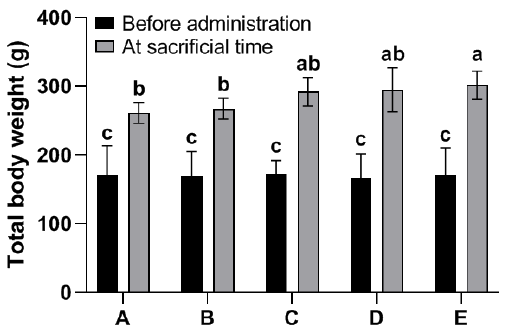
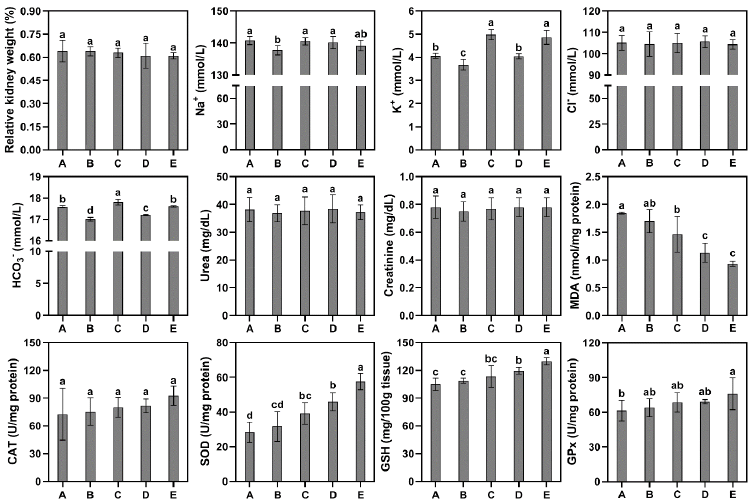
The effect of tea seed oil on serum electrolyte, urea, and creatinine concentrations
The group that received a dosage of 0.15 ml kg-1 TSO (B) exhibited a significant decrease in Na+ concentrations when compared to the control group. Nevertheless, there was no significant difference observed in the concentration of Na+ in the remaining groups compared to the control group. Likewise, the experimental group B demonstrated decreased levels of potassium (K+) and bicarbonate (HCO3–) ions. However, the administration of TSO at doses of 0.3 (C) and 0.6 (E) ml kg-1 resulted in higher levels of K+ and HCO3– compared to the control group. On the other hand, the concentrations of Cl– were not found to be significantly affected by TSO administration. Additionally, no significant differences were observed between the groups in terms of urea and creatinine concentrations (Fig. 2).
The effect of tea seed oil on antioxidant activity indicators
A notable decrease in the concentration of MDA was observed in groups C, D, and E in comparison to the control group. Although there was a small increase in catalase (CAT) activity in the groups administered with TSO, this increase did not reach statistical significance when compared to the control group. In contrast to the findings of MDA, the groups administered with TSO exhibited an elevation in the activity of superoxide dismutase (SOD) and glutathione peroxidase (GPx), as well as an increase in the content of reduced glutathione (GSH) in the kidney tissue. Nevertheless, the observed increase in SOD and GPx activity was found to be statistically significant only in groups C, D, and E while the elevation in GSH content was observed solely in group E (Fig. 2).
Discussion
The kidneys are vital organs that play a crucial role in maintaining homeostasis of bodily fluids, facilitating the removal of waste products from the circulatory system, and ensuring the proper balance of electrolytes. The examination of the impact of TSO on renal function can yield significant knowledge regarding its potential therapeutic or detrimental consequences. In order to achieve this objective, varying doses of TSO were administered to rats, encompassing a spectrum of concentrations ranging from low to high. This facilitated the examination of potential toxic effects that may arise as a result of the administration of TSO. The evaluated toxicological endpoints included the alterations in body weight and the kidney body weight index. Furthermore, various kidney function indices, indicators of oxidative stress, and measures of antioxidant status were examined.
The alteration of body weight can serve as a significant indicator of toxicity when evaluating the potential adverse effects of different substances, such as pharmaceuticals and chemicals [16]. Similarly, alterations in the weights of organs are regarded as significant indicators of toxicity and have the potential to offer valuable insights into the health implications of a particular substance. The presence of renal hypertrophy, whether absolute or relative, serves as an indication of exposure to nephrotoxic substances [17]. The findings of this study indicate that the administration of TSO does not result in any detrimental effects on the body weights and relative kidney weights of rats. In fact, it was observed that all groups under the administration of TSO exhibited a consistent increase in body weight over the course of the study. This may pertain to the potential orexigenic (appetite-stimulating) properties of TSO. There were no observed indications of toxicity among the animals belonging to different groups, and no instances of mortality were documented.
Electrolytes (sodium, potassium, calcium, magnesium, phosphate, and bicarbonates) play a crucial role in various physiological processes within the human body, encompassing the regulation of blood pressure, facilitation of muscle contractions, and transmission of nerve signals. The kidney plays a crucial role in maintaining optimal electrolyte homeostasis in bodily fluids. However, it is essential to acknowledge that imbalances can arise from various factors such as medication usage, dehydration, kidney ailments, and hormonal disorders [18]. The present results indicate that the concentrations of Na+ and Cl– in the groups administered with TSO were comparable to those observed in the control group. Although the serum of groups administered higher doses of TSO exhibited a notable elevation in concentrations of K+ and HCO3– ions, it is important to note that the concentrations of all electrolytes investigated remained within the normal range in the albino rat model [19]. Therefore, TSO might not have had a critical influence over electrolyte equilibrium in blood serum within the current experimental setting.
The measurement of urea is a crucial component in the assessment of kidney function [20]. In addition to urea, creatinine is another metabolic waste product that is excreted by the renal system. The generation of this compound arises from the breakdown of creatine phosphate within muscle tissue [21]. The serum creatinine levels are influenced by the rate of renal elimination, frequency of generation, and volume of distribution [22]. Improved kidney function is typically correlated with decreased levels of urea and creatinine. A previous report showed that receiving a pre-treatment of green tea extract (1 ml/100 g b.wt) before nicotine administration caused a notable reduction in serum levels of urea, creatinine, and uric acid in rats. Nevertheless, the present study revealed no statistically significant disparity in serum urea and creatinine levels between the groups administered with TSO and the control group. While the administration of TSO did not result in a reduction of urea and creatinine levels, the fact that these levels remained comparable to those of the control group suggests that TSO consumption did not adversely affect kidney function within the experimental conditions of the study.
Oxidative stress occurs in biological systems when there is an imbalance between the production rate of reactive oxygen species (ROS) and the body’s ability to remove them through the actions of antioxidants [23]. The deleterious impacts of oxidative stress on bodily organs are notably significant, with a particular vulnerability observed in the kidney. The high degree of vascularity and an increased rate of oxygen consumption are factors that contribute to the vulnerability of kidneys to this phenomenon [24]. The present investigation demonstrates that the administration of TSO led to a reduction in malondialdehyde (MDA) levels within kidney tissues. MDA serves as an indicator for the process of lipid peroxidation and the presence of oxidative stress. This particular substance is a result of the breakdown of polyunsaturated fatty acids through ROS [25]. Tea is known to possess bioactive compounds, specifically catechin polyphenols, that have the potential to scavenge ROS by directly binding to metal ions that play a role in the generation of these highly reactive molecules [26]. The currently observed decrease in levels of MDA may indicate that TSO has the ability to decrease oxidative damage to lipids and proteins, leading to reduced production of MDA in groups administered with TSO.
Superoxide dismutases (SODs) are a class of antioxidant enzymes characterized by the presence of metal ion cofactors. These catalysts facilitate reactions that cause the disproportionation of superoxide anion radicals into oxygen and hydrogen peroxide [27]. The findings of this study indicate that the administration of TSO, particularly at higher dosage levels, enhanced the animals’ ability to mitigate the effects of superoxide radicals by increasing the activity of SOD.
The findings additionally indicated that the administration of TSO at escalating dosages exhibited a favorable influence on the levels of glutathione (GSH) and glutathione peroxidase (GPx) in the kidney tissue of rat subjects. GSH is a significant antioxidant that assumes a prominent function in the neutralization of free radicals, which have the potential to disrupt regular cellular processes [28]. GPx is an enzyme that catalyzes the reduction of hydrogen peroxide and lipid hydroperoxides to less harmful substances, utilizing GSH as a reducing agent [29]. GPx is an integral component of the comprehensive cellular antioxidant defense mechanisms, which collaboratively function to safeguard renal cells against the detrimental effects of oxidative stress [30]. The asymmetry in GPx activity can have significant implications for renal health, rendering it a crucial variable to take into account when examining kidney injuries and exposures to nephrotoxic substances. The present study demonstrates a gradual and dose-dependent response in the observed elevation of GSH levels and GPx activity, as evidenced by the progressive increase from group B to E. When the levels of GSH are elevated, GPx utilizes the substantial abundance of its reducing agent, resulting in enhanced efficacy in the neutralization of peroxides [31]. As a result, the activity of GPx is enhanced, allowing it to operate more effectively in the reduction of peroxides and the neutralization of ROS. This measure guarantees enhanced safeguarding for cells and their constituent components.
Conclusion
The present study provides evidence to support the safety of tea seed oil (TSO) for consumption, as well as its potential health benefits, particularly in relation to kidney function and oxidative balance. The findings of this study indicate that there is a dose-response relationship, indicating that higher doses of TSO may have a more significant impact on kidney function and antioxidant status. It is recommended that further research be conducted to ascertain the acute and sub-acute toxicity of TSO in order to establish a definitive safe dose level.
References
1 | Kumar S, Paul S, Walia YK, Kumar A, Singhal P. Therapeutic potential of medicinal plants: a review. J. Biol. Chem. Chron. 2015;1(1):46-54. |
2 | Vaou N, Stavropoulou E, Voidarou C, Tsigalou C, Bezirtzoglou E. Towards Advances in Medicinal Plant Antimicrobial Activity: A Review Study on Challenges and Future Perspectives. Microorganisms. 2021;9(10):2041. DOI |
3 | Sofowora A, Ogunbodede E, Onayade A. The role and place of medicinal plants in the strategies for disease prevention. Afr. J. Tradit. Complement. Altern. Med. 2013;10(5):210-29. DOI |
4 | Zhang L, Ho CT, Zhou J, Santos JS, Armstrong L, Granato D. Chemistry and biological activities of processed Camellia sinensis teas: A comprehensive review. Compr. Rev. Food Sci. 2019; 18(5): 1474-95. DOI |
5 | Wang X, Zeng Q, Verardo V, del Mar Contreras M. Fatty acid and sterol composition of tea seed oils: Their comparison by the “FancyTiles” approach. Food Chem. 2017;233:302-10. DOI |
6 | Bolarinwa IF, Oke MO, Olaniyan SA, Ajala AS. A review of cyanogenic glycosides in edible plants. Toxicology–New Aspects to This Scientific Conundrum. 2016. DOI |
7 | Patel S, Lalwani K. The scary side of Ginkgo biloba is no match for an anesthesia superstar: Subdural: Ginkgo biloba. A Case Approach to Perioperative Drug-Drug Interactions. 2015;933-5. DOI |
8 | Tietz NW. Clinical guide to laboratory tests. In Clinical guide to laboratory tests. 1995;1096. |
9 | Bowers LD. Kinetic serum creatinine assays. The role of various factors in determining specificity. Clin. Chem. 1980;26:551-4. DOI |
10 | Richterich R, Kuffer H. The determination of urea in plasma and serum by a urease/Berthelot method. Klin. Biochem. 1973;11:553-64. |
11 | Goth L. A simple method for determination of serum catalase activity and revision of reference range. Clin. Chim. Acta. 1991;196:143-51. DOI |
12 | Marklund S, Marklund G. Involvement of the superoxide anion radical in the autoxidation of pyrogallol and a convenient assay for superoxide dismutase. Eur. J. Biochem. 1974;47:469–74. DOI |
13 | Banerjee B, Seth V, Bhattacharya A, Pasha S, Chakraborty A. Biochemical effects of some pesticides on lipid peroxidation and free-radical scavengers. Toxicol. Lett. 1999;107(1):33-47. DOI |
14 | Kinoshita C, Saze KI, Kumata S, Mastuki T, Homma S. A simplified method for the estimation of glutathione peroxidase activity and selenium concentration in bovine blood. J. Dairy Sci. 1996;79(9):1543–8. DOI |
15 | Okhawa H, Ohishi N, Yagi K. Assay for lipid peroxides in animal tissues by thiobarbitiuric acid. Anal. Biochem. 1979;95:351-58. DOI |
16 | Vaghasiya YK, Shukla VJ, Chanda SV. Acute oral toxicity study of Pluchea arguta boiss extract in mice. J. Pharmacol. Toxicol. 2010;6:113-23. DOI |
17 | Rajeh MB, Kwan YP, Zakaria Z, Latha LY, Jothy SL, Sasidharan S. Acute toxicity impacts of Euphorbia hirta L extract on behavior, organs body weight index and histopathology of organs of the mice and Artemia salina. Pharmacognosy Res. 2012;4:170-7. DOI |
18 | Tang C, Livingston MJ, Liu Z. Autophagy in kidney homeostasis and disease. Nat. Rev. Nephrol. 2020; 16: 489–508. DOI |
19 | Loeb WF, Quimby FW. The Clinical Chemistry of Laboratory Animals, 2nd ed. Philadelphia: Taylor & Francis USA. 1999. |
20 | Chinnappan SM, George A, Thaggikuppe P, Choudhary Y, Choudhary VK, Ramani Y, Dewangan R. Nephroprotective effect of herbal extract Eurycoma longifolia on paracetamol-induced nephrotoxicity in rats. Evid. Based Complementary Altern. Med.2019. DOI |
21 | Kashani K, Rosner MH Ostermann M. Creatinine: From physiology to clinical application. Eur J. Intern. Med. 2019;116:168-69. DOI |
22 | Mühlberg W, Platt D. Age-dependent changes of the kidneys: pharmacological implications. Gerontology. 1999;45(5):243-53. DOI |
23 | Sies H, Berndt C, Jones DP. Oxidative stress. Annu. Rev. Biochem 2017;86:715-48. DOI |
24 | Coppolino G, Leonardi G, Andreucci M, Bolignano D. Oxidative stress and kidney function: a brief update. Curr. Pharm. Des. 2018;24(40):4794-9. DOI |
25 | Morales M, Munné-Bosch S. Malondialdehyde: Facts and artifacts. Plant Physiol. 2019;180(3):1246-50. DOI |
26 | Bernatoniene J, Kopustinskiene DM. The role of catechins in cellular responses to oxidative stress. Molecules. 2018;23(4):965. DOI |
27 | Mirończuk-Chodakowska I, Witkowska AM, Zujko ME. Endogenous non-enzymatic antioxidants in the human body. Adv. Med. Sci. 2018;63(1):68-78. DOI |
28 | Adwas AA, Elsayed A, Azab AE, Quwaydir FA. Oxidative stress and antioxidant mechanisms in human body. J. Appl. Biotechnol. Bioeng. 2019;6(1):43-7. DOI |
29 | Pei J, Pan X, Wei G, Hua Y. Research progress of glutathione peroxidase family (GPX) in redoxidation. Front. Pharmacol. 2023;14:1147414. DOI |
30 | Piyarungsri K, Pusoonthornthum R. Changes in reduced glutathione, oxidized glutathione, and glutathione peroxidase in cats with naturally occurring chronic kidney disease. Comp. Clin. Path. 2016;25:655-62. DOI |
31 | Brigelius-Flohé R, Flohé L. Regulatory phenomena in the glutathione peroxidase superfamily. Antioxid. Redox Signal. 2020;33(7):498-516. DOI |
Cite this article:
Atanda, J., Yahaya, L., Nafiu, M., Mustapha, K., Ajewole, A. Unveiling the impact of tea (Camellia sinensis) seed oil on kidney function indices and antioxidant defense system in male albino rats. DYSONA – Life Science, 2023;5(1): 21-28. doi: 10.30493/dls.2023.422394